Contents
1. | Introduction | 3 | |
2. | Executive Summary | 3 | |
2.1. | Failures of Holliday et al. (2023) in the sections reviewed | 3 | |
2.2. | Conclusions | 6 | |
3. | The Younger Dryas Impact Hypothesis (YDIH) | 6 | |
4. | Important Concepts in Radiocarbon Dating | 7 | |
4.1. | Case Study 1: Gill et. al. (2009) | 7 | |
4.2. | Case study 2: O’Keefe et al. (2023) | 10 | |
4.3. | Summary of Section 4 and proper testing of the YDIH | 10 | |
5. | Rebuttal of HEA’s Section 1: Introduction | 11 | |
6. | Rebuttal of HEA’s Section 5: Inadequate Dating and Stratigraphic Context | 19 | |
6.1. | HEA’s Section 5.3. Deficient Dating of YDIH Sites | 19 | |
6.2. | HEA’s Section 5.4. Poorly Dated Platinum Anomalies | 28 | |
6.3. | HEA’s Section 5.5. Inconsistent dating of nanodiamond zones | 30 | |
6.4. | HEA’s section 5.6. Logical Lapses in Dating and Interpreting Usselo and Finow Soils | 31 | |
6.5. | HEA’s Section 5.7. Improved dating of Clovis sites and Clovis archaeology | 37 | |
6.6. | HEA’s Section 5.8. Radiocarbon simulations of the YDB | 40 | |
6.7. | Summary of HEA’s Sections 5.3-5.8 | 44 | |
7. | Rebuttal of other Sections of HEA | 45 | |
7.1. | The updated YD impact scenario | 45 | |
7.2. | Coherent catastrophism | 46 | |
7.3. | Microspherules | 49 | |
7.4. | Daulton et al.’s (2010) nanodiamond samples | 50 | |
7.5. | Platinum | 54 | |
7.6. | Summary of Section 7 | 57 | |
8. | Instances of Derision in HEA | 58 | |
8.1. | Summary of Section 8 | 61 |
1. Introduction
In an article of over 96,300 words, Holliday et al. (2023) (HEA) claim to have “comprehensively refuted” the Younger Dryas impact hypothesis (YDIH), which has been corroborated by scores of articles in dozens of peer-reviewed journals based on the discovery of a range of impact-related materials at more than 50 Younger Dryas boundary sites on five continents. To “comprehensively refute” a hypothesis so well-substantiated should require dispositive falsifying evidence that can be explained simply and in far fewer words. For example, it might be shown that samples claimed to be synchronous are clearly not, or that markers claimed to be extraterrestrial are instead clearly terrestrial. However, HEA present no new evidence of their own on any such possibility. Instead, the evidence they draw upon to support their case is frequently of low quality or fundamentally flawed. Moreover, their arguments are often distorted or misleading. Furthermore, they resort to unethical disparagement of, and ad hominem attacks upon, proponents of the hypothesis. Their approach is therefore a corruption of the scientific method and has been labelled “pseudoskepticism” (Powell, 2024).
The rhetorical tactic used in their prolix article is one well-enough known from creationists and other evolution deniers to have been given a name: the “Gish gallop” [Wikipedia, Gish gallop]. It is an attempt to overwhelm a debate opponent and prevent a coherent response by using a long list of arguments with little regard for their accuracy, relevance, or strength. This ruse makes it appear that the person using the Gish gallop has won the debate, simply because they have put forward more unaddressed points than their opponent, rather than because their arguments are more valid or compelling. Few have the time or energy to respond to, or even read, an article of nearly 100,000 words. The busy scientist is tempted simply to let it go. But that would allow rhetoric to triumph over evidence and allow virtual accusations of scientific misconduct to stand, corrupting the scientific record. It would also discourage researchers from pursuing a valid and potentially important hypothesis and funders from supporting it.
To counter a Gish gallop, one cannot and need not rebut every argument. Instead, it is sufficient to select a core set for analysis. If these are found to be flawed or unconvincing, then the entire article is called into question. We therefore focus in this rebuttal on Sections 5.3-5.8 of HEA, wherein they review evidence for the synchronous timing of the Younger Dryas boundary (YDB). If different boundary sites yield different radiocarbon ages, far outside their error bands, then an instantaneous cosmic event is unlikely to explain the evidence. We find that HEA fail to show that any YDB site is inconsistent with the suggested age of the YD impact and that this failure is caused by their misunderstanding of basic concepts in radiocarbon age-depth modelling, especially the treatment of uncertainty in experimental data.
In addition to a line-by-line rebuttal of Sections 5.3-5.8 of HEA, we also provide line-by-line rebuttals of their introductory section (Section 1 of HEA) and several other parts of their text.
To summarize, we review the following sections of HEA line-by-line:
Section 1: Introduction
Sections 5.3-5.8: Radiocarbon dating the YDB
Section 7: The updated YD impact scenario
Section 7: Coherent catastrophism
Section 4.1: Arlington Canyon confusion
Section 10: Microspherules
Section 11: Platinum
Before detailing our line-by-line counterarguments to HEA in later sections, we first present in the next section an executive summary of the main failings of HEA in the sections reviewed and our conclusions. Following this executive summary, we outline in Section 3 the Younger Dryas impact hypothesis (YDIH) itself, including recent updates to the hypothesis. We then discuss in Section 4 an issue that is central to much of the debate, namely the treatment of uncertainty in radiocarbon dating data. The importance of this issue requires that it is discussed before the detailed line-by-line rebuttals in later sections. To illustrate this issue, we focus in Section 4 on Gill et al. (2009) and O’Keefe et al. (2023) as case studies.
We finish our review of HEA by cataloging instances of disparagement that occur throughout their work. Normally, such remarks are proscribed in peer-reviewed journals. Indeed, Elsevier, who publish Earth-Science Reviews in which HEA is published, have a clearly stated publishing policy that should ensure such disparaging remarks are excluded from all their published articles. Unfortunately, breaches to Elsevier’s publishing policy by HEA appear to have evaded the attention of all those involved (the authors, reviewers and Editor). Powell (2024) links these failures with an increasingly vehement trend in the disparagement of YDIH proponents that has the apparent aim of suppressing the hypothesis.
2. Executive Summary
2.1. Failures of Holliday et al. (2023) in the sections reviewed
In Sections 5 through to 7 of this report, we provide a detailed critique of several sections of HEA’s review of the YDIH, highlighting numerous errors in their presentation. We show that HEA does not contain what we regard as a refutation of the YDIH, but rather a disagreement over the interpretation of studies by YDIH proponents. Nor does the HEA article contain any new data, much less falsifying data.
In the summary below, we list the major errors encountered only in the sections of HEA reviewed here. Many of the issues highlighted in this list were already made in previous reports by YDIH proponents. It is not clear why they have been effectively ignored by HEA. Our conclusions follow this list.
HEA frequently avoid addressing valid tests of the YDIH by claiming such tests represent circular reasoning. As shown in our Section 4, their claims are false. If valid tests of the YDIH are routinely rejected on this basis then HEA’s viewpoint becomes a self-fulfilling prophecy. Thus, HEA lacks the basic logic required for proper testing of the YDIH.
HEA fail to acknowledge a basic underpinning dogma of science, which is “model efficiency”, “parsimony”, or “explaining power”, sometimes paraphrased as “Occam’s razor” (Mcfadden, 2023). Science can be distinguished from pseudoscience through use of this principle. Our conclusions are based on this fundamental scientific principle. That is, a series of highly correlated impact events distributed across at least four continents provides an explanation for the very strong correlation between a widespread layer of apparently synchronous geochemical impact-related markers and the onset of YD cooling, the end of Clovis culture and a cluster of megafaunal extinctions. The YD impact event is also reasonably probable, had been predicted for decades, and can create the evidence observed.
HEA fail to acknowledge that it is standard practice to update a hypothesis when new information is obtained, and that such updates supersede older definitions of the hypothesis. This is a mark of good science, not a fault.
HEA consistently fail to test the HEA according to its own predictions, i.e. they frequently criticize ideas that are not the YDIH or correspond to early versions of the YDIH without taking any updates into account. Their criticism based on such “strawman” arguments is therefore confused. This failure takes many forms, including;
HEA consistently fail to acknowledge that the YDIH is scientifically defined first by Firestone et al. (2007) and updated most recently in Wolbach et al. (2018a, b) with a detailed astronomical impact model presented in Moore et al. (2023) after HEA was published. Instead, HEA give apparently equal weighting to ideas proposed in a wide range of sources, many not even authored by YDIH proponents. Yet HEA must be aware of the updates in Wolbach et al. (2018a, b) because these reports form the basis of criticism for Holliday et al. (2020).
HEA consistently fail to recognise that the preferred impact scenario, reiterated by Wolbach et al. (2018a, b) and again by Moore et al. (2023), consists of a swarm of comet fragments that impacted an entire hemisphere of Earth. Many of these impacts are expected to be low-altitude airbursts, but ground impacts are not yet ruled out.
A basic tenet of the YDIH is that the YDB debris layer represents a narrow period and can be used as a datum. Due to its long-lived atmospheric lifetime and transport, probably the most widespread kind of impact proxy is the platinum anomaly which has been found in the GISP2 ice core and at many YDB sites. HEA consistently fail to use the YDB as a chronological datum against which archaeological, paleontological and paleoenvironmental signals can be compared. This applies especially to Clovis artifacts, megafaunal remains and other environmental indicators such as charcoal and pollen.
The date of the YD impact is determined via radiocarbon dating to be 12,785-12,885 cal BP using the latest radiocarbon calibration curve (see Cheng et al. (2020)). In the GISP2 ice core the YD onset is determined by the platinum anomaly at 12,825 ± 5 BP using the GICC05 chronology (see HEA). This ice core age scale has a maximum counting error of ± 140 years at this point. Instead of using these ages/dates, HEA frequently use alternative definitions for the YD onset. For example, HEA claim the YD onset is known from analysis of the deuterium excess signal in the NGRIP ice core (Steffensen et al., 2008). But this definition is contentious.
HEA frequently infer that the YDIH predicts the instantaneous extinction of megafauna or the end of Clovis precisely at the YD onset. Instead, the YDIH proposes the YD impact had a significant effect on megafaunal populations and the Clovis culture.
HEA consistently fail to acknowledge that the YDIH does not live or die by its predictions of secondary effects. For example, if it is found eventually that the Clovis decline was not triggered by the YD impact, this does not rule out the possibility the impact had a significant influence on megafaunal populations.
HEA frequently misuse confidence intervals, error bars and uncertainty estimates. There are many examples even in the few sections we review, mostly relating to misuse of radiocarbon data. For example;
HEA fail to acknowledge that the intrinsic uncertainty in individual radiocarbon measurements is not a good predictor for the true sample age uncertainty. This is shown explicitly in this report for several data sets relevant to the debate.
HEA rely heavily on Meltzer et al. (2014) in Section 5 of their report to dispute the synchroneity of the YDB across several continents. Yet the linear age-depth models generated by Meltzer et al. (2014) omit confidence intervals for their linear coefficients and they instead relied only upon median calibrated ages in their Table 3. Therefore, their linear models are not scientific and their conclusions are unsupported. We show that inclusion of the correct confidence intervals would have nullified Meltzer et al.’s (2014) claims.
HEA do not recognize that age-depth models must be created using uncalibrated radiocarbon data. These models can then be converted to a calibrated age scale in a final step.
HEA claim with absolute certainty the charcoal-rich layer known as the Ussello/Finow horizon in western Europe is time transgressive, citing many radiocarbon measurements from a wide range of sites. However, HEA misunderstand the nature of variance in radiocarbon data sets. In fact, the radiocarbon data from the charcoal-rich Ussello/Finow horizon is consistent with a synchronous event.
HEA support the conclusions of O’Keefe et al. (2023) regarding the megafaunal extirpation near the Rancho La Brea tar pits which is claimed “almost certainly” to take place before the YD onset based on analysis of radiocarbon data. However, this “almost certain” claim is inconsistent with the 1-sigma confidence interval used. At 2-sigma, this extirpation is consistent with the YDIH.
HEA frequently demand evidence of YDIH proponents that is unnecessary or unreasonable. For example;
HEA frequently demand evidence for ground impacts when such impacts are not required to explain the evidence. Nevertheless, ground impacts are not ruled out by the YDIH.
HEA frequently demand that only precise dates for the YDB are acceptable. This is unreasonable. Instead, we must deal with the evidence that is found, not the evidence we would like to find. Even relatively imprecise dates for the YDB can be useful, as demonstrated in our response to HEA’s section 5.3 in our Section 6.
HEA frequently take seemingly inconsistent positions. For example;
HEA claim that microspherule evidence alone cannot be used to establish an ET impact event. However, this contradicts the review by French and Koeberl (2010) that suggests that this is possible (note that C. Koeberl is a co-author of HEA). Moreover, HEA quote French and Koeberl (2010) as follows; “Like other impact melts, droplet spherules generally preserve no evidence of shock processes or of their original ultrahigh-temperature origin….” It is especially noteworthy that HEA terminate their quote at this point because the next line in French and Koeberl (2010) reads “There are rare exceptions: inclusions of lechatelierite, coesite, and shocked zircon, which establish an impact origin directly…”. Lechatelierite has been found at several YDB sites.
HEA claim that coherent catastrophism “is a speculative hypothesis that is unsupported by observational data and inconsistent with the cratering record” and call it “a preposterous fringe idea”. Yet they cite no evidence to support this view and fail to acknowledge that the giant comet origin model for the Taurid meteor stream is the working model for cometary scientists. This model is consistent with coherent catastrophism, i.e. the temporary trapping of comets from the outer solar system in Earth-crossing orbits. Later in the same section, HEA claim that there is some truth to the concept of coherent catastrophism, but they disagree with an “extreme version” of it, without defining what they mean or providing any supporting evidence. HEA further claim that results of the 2019 observational campaign on the Taurid resonant swarm contradict this model. However, HEA fail to acknowledge that this campaign never took place as intended and no papers have been written about it. Moreover, they also fail to mention that the existence of the Taurid meteor swarm was already confirmed by earlier observational campaigns (see Section 7.2)
HEA claim that the GISP2 platinum signal could be generated by the Cape York meteorite without acknowledging that this claim is entirely speculative (Boslough 2013; Beech et al. 2020). However, they also claim the platinum signal requires a ground impact (without providing any supporting evidence) but fail to acknowledge that the Cape York meteorite is not associated with any crater.
HEA continue to support the conclusions of Daulton et al. (2010) regarding the irreproducibility of the Arlington Canyon nanodiamond evidence. However, it is clear from their own words, photographs of stratigraphic sections, and the map coordinates in Scott et al. (2010) that Daulton et al. (2010) did not sample the same YDB site as Kennett et al. (2009) nor did they collect samples likely to contain nanodiamonds. Indeed, one site they sampled was over 7 km away from the site sampled by Kennett et al. (2009). HEA avoid directly addressing this issue by consistently conflating the nanodiamond evidence in Daulton et al. (2010) with nanodiamond evidence in later work by Daulton et al. (2017).
HEA mistreat the nanodiamond evidence from Bull Creek provided by Kennett et al. (2009) by shifting it upwards by one cell in their Table 5. They then criticize YDIH proponents by claiming the nanodiamond evidence provided by Kennett et al. (2009) and Bement et al. (2014) is inconsistent. In fact, the only inconsistency relates to the units used by Bement et al. (2014) which might be a typo.
HEA claim that an ammonium abundance and a platinum abundance in the GISP2 ice core are not synchronous with the YD onset. But this view is based on the misleading presentation of this data in Petaev et al. (2013). HEA ignore the correct plotting of this data highlighted by Sweatman (2021) and they ignore higher resolution data plotted in Wolbach (2018a).
HEA claim that a sudden “impact winter” can be ruled out through analysis of low-resolution palaeoclimate data. However, only high-resolution sub-annual data can be used to investigate this issue. HEA’s argument that the suggested impact winter must have lasted for several years is based on an understanding of volcanic eruptions, not ET impacts.
HEA frequently conflate the cluster of megafaunal extinctions around the YD onset with other megafaunal extinctions over the last glacial cycle. The YDIH says nothing about these other extinction events.
HEA mistakenly claim that mixed assemblages of Clovis and other cultural artifacts contradict the YDH. But the YDIH makes no claims about other cultures and taphonomic mixing is commonplace.
HEA fail to acknowledge or understand inherent problems in the work of Jorgeson et al. (2020, 2022) on the synchroneity of the YDB across several continents which were pointed out by Sweatman (2021). More problems are identified in this rebuttal.
HEA frequently use derisory terms and language which have no place in a scientific report. They also frequently make baseless, defamatory accusations. Consequently, Powell (2024) labelled their work as “pseudoskeptcism”.
2.2. Conclusions
Our detailed analysis of several sections of HEA revealed the major errors summarized above. But these sections of HEA contain a much longer list of more minor errors, often in the form of misleading statements. While we have only provided a detailed critique of some sections of HEA in this report, we find that the remainder of HEA is quite similar. It is therefore clear to us that HEA is a Gish gallop, i.e. a long series of weak arguments designed to overwhelm an opponent.
This explains the strong inverse correlation between the length of HEA and the strength of its arguments. That is, this inverse correlation is expected because to make their arguments HEA must repeatedly distort the facts, and this takes significant effort. If the YDIH were false it should be easy to refute in far fewer words.
HEA claim to refute the YDIH, but their claim should be rejected because;
They fail to provide a reasonable alternative explanation for the abundance of geochemical impact proxies with their specific characteristics at 58 YDB sites (and counting) on at least four continents. All 58 sites show abundance peaks in up to six impact proxies, such as exotic microspherules, platinum and nanodiamonds, that are consistent with an impact date of 12885-12785 cal BP (using the latest radiocarbon calibration curve, see Cheng et al., 2020). The most parsimonious explanation is that they have a common source - the YDB impact event. Since this evidence has been reproduced by independent researchers many times, the possibility that it is all mistaken, manufactured, or not really there is absurd.
They fail to show that the age of any YDB site is inconsistent with the suggested age of the YD impact. We have shown here that the ages of many of these sites are so close to each other within error bounds that the only reasonable interpretation is that a singular impact event or series of highly correlated events took place.
They fail to show that Clovis artifacts are consistently found above the YDB. Indeed, they even fail to show that any Clovis artifacts are found above the YDB.
They fail to show that a cluster of megafaunal extinctions is not strongly correlated with the YDB and the YD onset. Indeed, multiple independent studies show a cluster of megafaunal extirpations or extinctions synchronous with the YD onset (within dating uncertainty), followed by further extinctions, just as expected (Boulanger et al. 2014; Villavicencio et al. 2015; Stewart et al. 2021; O’Keefe et al. 2023). This is partly due to, among other mechanisms, the survival in refugia of small megafaunal populations that later went extinct.
3. The Younger Dryas Impact Hypothesis (YDIH)
The Younger Dryas impact hypothesis is first described in Firestone et al. (2007);
“We propose that one or more large, low-density ET objects exploded over northern North America, partially destabilizing the Laurentide Ice Sheet and triggering YD cooling. The shock wave, thermal pulse, and event-related environmental effects (e.g., extensive biomass burning and food limitations) contributed to end-Pleistocene megafaunal extinctions and adaptive shifts among PaleoAmericans in North America.”
Furthermore, in their text we read;
“A number of impact-related effects most likely contributed to the abrupt, major cooling at the onset of the YD and its maintenance for ~1,000 years. Cooling mechanisms operating on shorter time scales may have included … (ii) atmospheric injection of nitrogen compounds (NOx), sulfates, dust, soot, and other toxic chemicals from the impact and widespread wildfires (46), all of which may have led to cooling by blockage of sunlight…. The largest potential effect would have been impact-related partial destabilization and/or melting of the ice sheet…. The longer-term cooling effects largely would have resulted from the consequent weakening of thermohaline circulation in the northern Atlantic (54), sustaining YD cooling for ~1,000 years.”
Since this initial proposal, the geochemical evidence gathered suggests a more widely distributed event than implied above. A major update to the YDIH was therefore provided by Wolbach et al. (2018a), which also has many of the same co-authors as Firestone et al. (2007). We read in their section “Extended YD impact theory”;
“On the basis of evidence from the YDB, known impact events, and nuclear detonations, here we update the YDB impact theory. A giant, ≥100-km-diameter comet entered an Earth-crossing orbit in the inner solar system and began a cascade of disintegrations (Napier 2010). Numerous cometary fragments from the debris stream entered Earth’s atmosphere ~12,800 y ago and detonated above and/or collided with land, ice sheets, and oceans across at least four continents in the Northern and Southern Hemispheres (Firestone et al. 2007; Napier 2010). … The airburst/impacts collapsed multiple ice dams of proglacial lakes along the ice-sheet margins, producing extensive meltwater flooding into the Arctic and North Atlantic Oceans (Teller 2013; see Kennett et al. 2018 for summary and references). … The massive outflow of proglacial lake waters, ice-sheet meltwater, and icebergs into the Arctic and North Atlantic Oceans caused rerouting of oceanic thermohaline circulation. Through climatic feedbacks, this, in turn, led to the YD cool episode (Broecker 1997; Teller 2013; Kennett et al. 2018). … The radiant and thermal energy from multiple explosions triggered wildfires that burned ~10% of the planet’s biomass, producing charcoal peaks in lake/marine cores that are among the highest in 368,000 y (Wolbach et al. 2018a, b). This widespread biomass burning generated large amounts of long-lived, persistent AC/soot that blocked nearly all sunlight, rapidly triggering impact winter that transitioned into the YD cool episode (Wolbach et al. 2018a, b). … Climate change, wildfires, and related environmental degradation contributed to the late Pleistocene megafaunal extinctions and human cultural shifts and population declines (Firestone et al. 2007; Anderson et al. 2011; Wolbach et al. 2018a, b; this study).”
A similar account is provided in Wolbach et al. (2018b), which also has many of the same co-authors as Firestone et al. (2007). Thus, since 2018 the favored impact model involves a multitude of comet fragments from a previously disrupted giant comet dispersed across several continents. Moore et al. (2023), published after HEA, continues to favour the fragmented comet scenario. Some fragments may have been sufficiently large to produce ground impacts. Together, these are proposed to have triggered extensive wildfires as well as massive flooding sufficient to alter major ocean currents leading ultimately to the Younger Dryas cooling, many megafaunal extinctions, and significant changes to human populations and cultures. The giant comet scenario proposed is consistent with a process known as ‘coherent catastrophism’ which is thought to be responsible for creation of the Taurid meteor stream (Asher et al. (1994); Steel and Asher (1996); Napier (2019)). Note that neither version of the YDIH above posits an instantaneous megafaunal extinction or major human cultural extinction or depopulation event.
4. Important Concepts in Radiocarbon Dating
The YDB is ~12,850 years old (Kennett et al., 2015a), and therefore radiocarbon should be a suitable method for dating it. This method has proven revolutionary in many fields, but it has several inherent problems that require special care in determining whether two radiocarbon dates are likely to be synchronous. We show later in our Section 6 through line-by-line rebuttal of HEA’s Sections 5.3 – 5.8 that HEA fundamentally misunderstand such important concepts.
The key point here is that an instantaneous and widely distributed event (e.g. the Younger Dryas (YD) impact), due to the inherent uncertainty in radiocarbon dating, can appear to take place over several thousand years if radiocarbon dates from different sites are taken at face value, as HEA do. Stated more technically, HEA fail to understand that the intrinsic radiocarbon measurement uncertainty is, in general, not a good proxy for the true sample age uncertainty. To illustrate this point, we discuss two case studies below that examine the radiocarbon data and conclusions of Gill et al. (2009) and O’Keefe et al. (2023), which are both cited by HEA as evidence against the YDIH.
4.1. Case Study 1: Gill et al. (2009)
Gill et al.’s (2009) highly cited paper concerns the end-Pleistocene megafaunal extinctions and claims to contradict the YDIH. They use sediment cores from Lake Appleman, Indiana, to infer environmental changes near the lake around the Younger Dryas period. Figure 1 shows their key results.
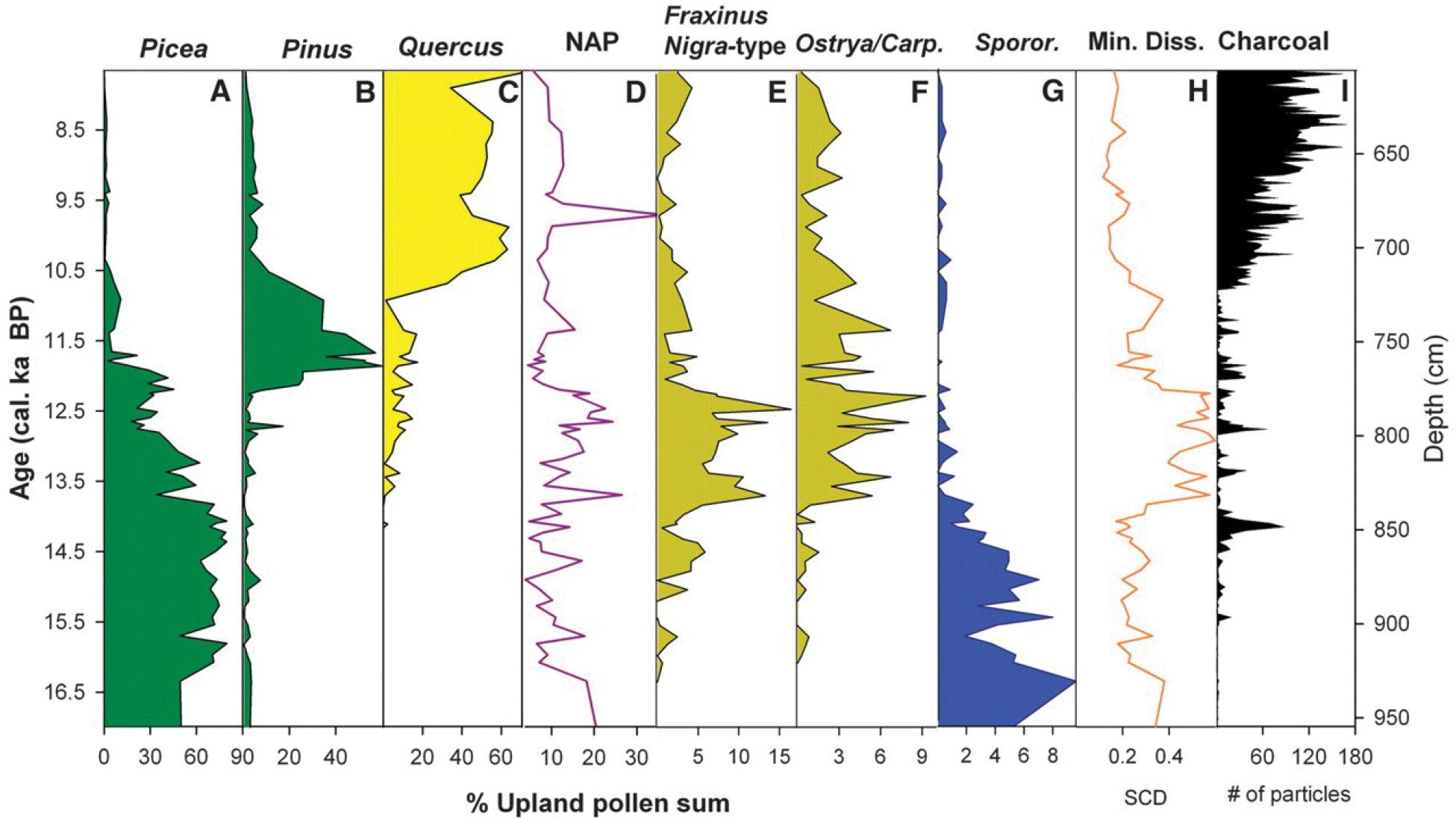
Data measured from a sediment core taken from Appleman Lake, Indiana, by Gill et al. (2009).
Note that a major charcoal-producing event occurs just above 850 cm and is correlated with the onset of an extended feature in “Min. Diss.”, which is used by Gill et al. (2009) as a proxy for aggregated vegetation change around the lake, and with a significant decline in “Sporor.”, which is used by Gill et al. (2009) as a proxy for megafaunal populations near the lake. The Depth-Age axis of this plot is shown without any uncertainty and is deduced via linear regression of radiocarbon dating measurements taken from the same core (see Figure 2). In their abstract Gill et al. (2009) conclude;
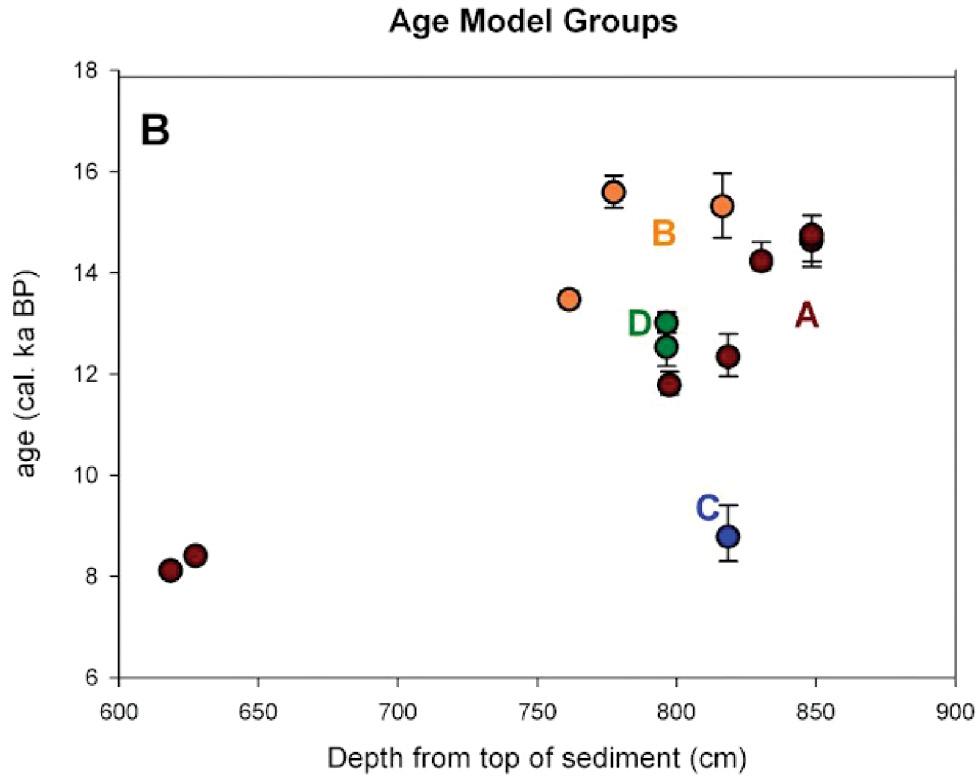
Part of Figure S1 from the appendix of Gill et al. (2009). This data is used to construct their age-depth model.
“Megafaunal populations collapsed from 14,800 to 13,700 years ago, well before the final extinctions and during the Bølling-Allerød warm period. Human impacts remain plausible, but the decline predates Younger Dryas cooling and the extraterrestrial impact event proposed to have occurred 12,900 years ago.”
Note that this statement is made without stating any uncertainty in the ages quoted.
Figure 2 shows the radiocarbon data from which Gill et al. (2009) deduced their age-depth model. Clearly, the scatter in the radiocarbon data in Figure 2 is far larger than the error bars shown which reflect the intrinsic uncertainty in the radiocarbon measurements themselves. Indeed, the scatter in the data covers nearly 6,000 years while some of the intrinsic measurement errors are only a few hundred years (some are even smaller than the symbol size). This is obviously inconsistent and demonstrates that the error bars provided are almost certainly too small. Nevertheless, if similar measurements were taken individually from a correlated feature at a wide range of sites (such as the Younger Dryas boundary), since the scatter in radiocarbon dates can be much greater than the intrinsic measurement error it might be concluded that the feature was generated gradually over a wide geographical range when, in fact, a synchronous event remains a realistic possibility.
This effect is quite common in radiocarbon data sets. It is often caused by non-radiogenic processes, such as the ‘old wood’ effect or leaching by ‘young water’, that can affect the apparent age of a sample. Additional uncertainty is created by the relative movement of objects within sediments, e.g. via bioturbation or other ground disturbances like flooding.
However, these are ‘known unknowns’. There could be many more ‘unknown unknowns’, i.e. processes of which we have no knowledge, yet which significantly affect the radiocarbon measurement of a sediment sample or its position within a sediment column. It follows that single radiocarbon measurements of sediments are unreliable for estimating their age since, in principle, they cannot provide a good estimate of the true sample age uncertainty by themselves. Instead, a suite of measurements from different sediment depths should be used. The more samples that are taken, the better the estimate of the true sample age uncertainty. Age-depth models can then be constructed, provided a proper account of the uncertainty in the set of measurements is made.
Often, age-depth models are constructed simply by linear regression, or line fitting, using the method of least squares. In this case there are two main options; to either use the intrinsic measurement error by performing a weighted fit, or to estimate the measurement error self-consistently by performing an unweighted fit. In Figure 2 above, as the scatter in the data is far greater than the intrinsic measurement error an unweighted regression method should be used. Gill et al. (2009) do not state whether they perform a weighted or unweighted fit. Regardless, the main problem with their analysis is that they did not report the uncertainty in their fitted linear coefficients which is contrary to best practice in science. By not reporting such error estimates it is impossible to draw reliable conclusions from their work. Nevertheless, as shown by the quote above, Gill et al. (2009) concluded with unqualified certainty that the megafaunal collapse pre-dated the Younger Dryas.
To provide this confidence interval it is necessary to perform linear regression on the raw C14 radiocarbon dates rather than the calibrated radiocarbon data. Although no statement is provided by them, it appears Gill et al. (2009) used the latter. To see why this is important consider an example where 1 million radiocarbon measurements were made, and each was converted to a calendar age using a calibration curve. In this case, the line of best fit to the calendar age data would likely have only a tiny uncertainty due to the very large number of data points. Indeed, the regression uncertainty would likely be far less than the radiocarbon calibration uncertainty. This effect is due to the ‘Law of large numbers’, which is of central importance in data analysis, statistics and science generally. But this procedure is erroneous because radiocarbon calibration uncertainty is immutable - it is simply not possible to eliminate or reduce radiocarbon calibration uncertainty this way. Thus, linear regression must be performed with the raw C14 radiocarbon data. Then, all this data, i.e. the line of best fit plus its uncertainty estimates, should be converted to a calendar age in a final step through proper convolution of the uncertainty in the fit to the raw C14 radiocarbon data with the calibration curve uncertainty.
Sweatman (2021) already described such flaws in Gill et al. (2009);
“… their reasoning is flawed because they fail to properly account for the uncertainty in their age-depth model. Even casual inspection of their radiocarbon measurements in their supplementary information (see Fig. 9b) suggests this charcoal layer is not inconsistent with a Younger Dyas age, and, moreover, it appears coeval with the onset of a period of dramatic change in vegetation around the lake apparent between 850 and 780 cms whose duration correlates well with the Younger Dryas period. Gill et al. (2009) essentially ignore this strong correlation, as well as the inherent uncertainty in their radiocarbon measurements, in determining their age-depth model. This is an important observation because their highly-cited work is often used to refute the impact theory. Rather, this work could be viewed as strongly supporting it.”
HEA criticize Sweatman’s (2021) analysis as “circular reasoning” (their Table 8) but they do not explain how it is circular. It is not at all clear to us how Sweatman’s reasoning can be viewed as circular, but perhaps HEA unreasonably reduce his reasoning to the following circular argument: 1) “the YDIH is likely correct, therefore Gill et al.’s evidence is best explained by the YDIH” and 2) “Gill et al.’s evidence is best explained by the YDIH, therefore the YDIH is likely correct”. While these two statements by themselves are circular, this does not mean that either is incorrect. That is, circular reasoning is not a logical fallacy. Instead, the problem with circular reasoning is that it isolates the reasoning from other evidence that may be relevant. Thus, statements involved in circular reasoning are impossible to prove or disprove by themselves. If the above circular reasoning is indeed the basis of HEA’s objection, then it is not a fair representation of Sweatman’s position at all. In fact, Sweatman’s position is actually the following, and this should have been self-evident to HEA: 1) “The YDIH is likely correct because of the mass of evidence that supports it, therefore we should investigate whether Gill et al.’s evidence can be explained by the YDIH”, 2) “Since Gill et al.’s evidence is best explained by the YDIH when the uncertainty in the age-depth scale is taken into account, it adds further support to the YDIH”. This sequence of reasoning is clearly not circular because it references other evidence. In fact, Sweatman’s argument is based on the principle of parsimony, also known as Occam’s razor, which is the fundamental basis of science (McFadden, 2023). Sweatman considers both the correlation within Gill et al.’s (2009) evidence, i.e. between ‘Min. Diss.’, ‘Sporor.’ and ‘Charcoal’, as well as the correlation between Gill et al.’s (2009) evidence and all the other evidence relating to YDIH, and attempts to find a consistent and relatively simple explanation. This is good science.
Therefore, Sweatman’s (2021) explanation should be preferred to Gill et al.’s (2009) explanation for their data. HEA’s suggestion of circular reasoning unreasonably prevents discussion of Sweatman’s hypothesis. In fact, by claiming his reasoning is circular, HEA fail to use fundamental underpinning concepts in science, i.e. hypothesis testing and parsimony. Later, we will see this rhetorical device used many times by HEA. It appears their strategy is to use the claim of circularity at every opportunity to avoid discussing evidence which supports the YDIH.
Of course, there is an easy way to test Sweatman’s (2021) hypothesis: if it is correct, then geochemical indicators of an impact, such as an abundance of platinum, microspherules or nanodiamonds, should be found within the charcoal layer at Appleman Lake at 850 cms. Until such tests have been performed, Sweatman’s (2021) hypothesis should be preferred. At the very least, the work of Gill et al. (2009) does not refute the YDIH.
4.2. Case study 2: O’Keefe et al. (2023)
A few weeks after HEA appeared, O’Keefe et al. (2023) reported that several late Pleistocene megafaunal extirpations near the Rancho La Brea tar pits in California are consistent with a synchronous event. Since this event also appears to correlate with extensive wildfires, as evidenced by a charcoal layer in nearby lake sediments, they concluded the megafaunal decline and wildfires were likely driven by human activity in the region. They do not mention or cite any literature pertaining to the YDIH, which even if rejected is certainly relevant.
Figure 3 shows their main result. They state;
“All extinct mammals dated in this study have last occurrence dates older than 13.00 ka, with a modeled extirpation time estimate across all taxa of 13.07 to 12.89 ka [using the Gaussian-Resampled Inverse-Weighted McInerney (GRIMW) extinction estimator; Table 1], placing the all-taxon extirpation almost certainly before the onset of the Younger Dryas (12.87 ± 0.03 ka) (14).”
Radiocarbon dated YDB sites. The radiocarbon date “cal BP” uses the 2013 calibration with uncertainty quoted at 2-sigma (2σ). “Kennett” refers to Kennett et al. (2015a). The remaining YDB sites are not dated directly and so are excluded from this list. Watcombe Bottom, UK, is the average of three dated local sites (see Kinzie et al. (2014) for the source reference).
Country | Site | cal BP | 2σ | Refs |
---|---|---|---|---|
Canada | Lake Hind | 12745 | 360 | Kennett |
Chile | Pilauco | 12770 | 320 | Pino et al. (2019) |
Chile | El Salto | 13275 | 315 | Pigati et al. (2012) |
Venezuela | Mucunuque | 12787 | 60 | Mahaney et al. (2023) |
EU, Belgium | Lommel | 12735 | 1580 | Kennett |
EU, Czech | Stara Jimka site | 12757 | 218 | Kletetchka et al. (2018) |
EU, Germany | Lingen | 12735 | 170 | Kennett |
EU, Netherlands | Aalsterhut | 12780 | 70 | Kennett |
EU, Netherlands | Ommen | 12750 | 1120 | Kennett |
EU, Spain | Santa Maira | 12785 | 580 | Kennett |
EU, UK | Watcombe Bottom | 12950 | 180 | Kinzie et al. (2014) |
Mexico | Lake Cuitzeo | 12897 | 375 | Kinzie et al. (2014) |
Syria | Abu Hureyra | 12825 | 110 | Kennett |
US, AZ | Murray Spgs | 12740 | 460 | Kennett |
US, CA | Arlington Cyn | 12805 | 110 | Kennett |
US, CA | Daisy Cave | 12730 | 640 | Kennett |
US, CA | Talega | 12860 | 300 | Kennett |
US, CA | Dove Spring | 12400 | 250 | Pigati et al. (2012) |
US, CO | Lindenmeier | 12775 | 360 | Kennett |
US, MI | Gainey | 12300 | 2460 | Wittke et al. (2013) |
US, MO | Big Eddy | 12770 | 170 | Kennett |
US, MT | Indian Creek | 12750 | 850 | Kennett |
US, NC | Barber Creek | 12865 | 1070 | Kennett |
US, NC | Squires Ridge | 12400 | 1200 | Moore et al. (2017) |
US, NM | Blackwater Draw | 13210 | 690 | Kennett |
US, OH | Sheriden Cave | 12840 | 240 | Kennett |
US, OK | Bull Creek | 12840 | 150 | Kennett |
US, PA | Melrose | 12255 | 4810 | Kennett |
US, SC | Blackville | 12820 | 2160 | Kennett |
US, SC | Topper | 12785 | 370 | Kennett |
However, their statement is internally inconsistent since the phrase “almost certainly” is applied to a confidence interval of only 1-sigma (66.7%). At the level of 2-sigma (95.6%) this date range overlaps the proposed age of the YDB. Therefore, their results are consistent with the YDIH and contradict the conclusion of Gill et al. (2009) that the megafaunal extinction predated the YD onset.
HEA repeat O’Keefe et al.’s (2023) conclusion in Section 3.2 of their work; “Further the complexity of extinction is demonstrated by data from Rancho La Brea, California (O’Keefe et al., 2023) where seven species were extirpated before the onset of the YDC.” Note the absolute certainty in this statement which is inconsistent with the evidence.
Both O’Keefe et al. (2023) and Gill et al. (2009) focus on evidence for a local extirpation. But Gill et al. (2009) extrapolate their local extirpation to a hemispheric extinction. But, of course, species may experience local extirpation for many reasons without suffering large-scale total extinction. Thus, neither study contradicts the YDIH as HEA claim. On the contrary, they both offer good evidence in support of the YDIH.
4.3. Summary of Section 4 and proper testing of the YDIH
Both Gill et al. (2009) and O’Keefe et al. (2023) show how uncertainty in radiocarbon dating data has been misapplied against the YDIH. HEA cite both studies as evidence counting against the YDIH, when in fact both studies offer good support for the impact hypothesis. Essentially, HEA dismiss evidence that supports the YDIH through a false claim of circularity and thereby avoid discussing it.
As already explained by Sweatman (2021), to properly test the YDIH research must compare the archaeological context, such as megafaunal remains and Clovis artifacts, to the YD boundary (YDB). Any study that fails to do this, or which does not properly take uncertainties in radiocarbon dating into account, cannot be used to refute the YDIH.
Petaev et al. (2013) discovered a platinum signal in the GISP2 ice core which is apparently synchronous with a sudden drop in temperature at that location and attributed it to an ET event. Because of its ~ 20-year duration in the ice, they remarked that the platinum signal should be a global atmospheric anomaly. Moore et al. (2017) later found a platinum anomaly at 11 YDB sites exactly where it was expected based on either radiocarbon dating, the position of other impact proxies, or the position of other archaeological indicators such as terminal Clovis points. To date, a consistent platinum anomaly has been found at 19 more YDB sites. Arguments in Section 6 of this work suggest this excess platinum, along with the other impact proxies invariably found with it, is very likely synchronous at each site where it is found.
This layer of impact proxies, known collectively as the YD boundary (YDB), is found on at least five continents, although it is not known to be perfectly continuous across this wide area. The YDB is often found at the base of a YD black mat which can serve as a good guide to its location. The black mat, first reported by Haynes (2008), is present at about half of YDB sites and usually represents environmental changes due to abrupt impact-related YD climate change, combined with YDB impact proxies.
We recommend that this platinum anomaly be used as a chronostratigraphic datum to correlate the YDB at other archaeological and paleontological sites with the YDB in the GISP2 ice core. In fact, since Moore et al. (2017) was published, it has been used in precisely this manner in studies on three different continents (Pino et al. 2019; Moore et al. 2019; Thackeray et al. 2019), and most recently at Wakulla Springs, Florida (Moore et al. 2023).
5. Rebuttal of HEA’s Section 1: Introduction
In the following, we respond line-by-line to HEA’s Section 1: Introduction. Quotes from HEA are labeled and non-italicized; our comments are indented in italics.
HEA’s Section 1: Introduction
HEA: The Younger Dryas impact hypothesis (YDIH) is a collection of ideas proposed to explain terminal Pleistocene environmental change across North America and other continents at the onset of the Younger Dryas (YD) stadial and the beginning of the YD Chronozone (YDC) (Section 2).
This is misleading. The YDIH is not a “collection of ideas” and no evidence is provided to justify this statement. Instead, it is a coherent hypothesis. See our Introduction above for clear statements about the YDIH, including an update made by many of the same authors as the original hypothesis which HEA acknowledge.
HEA: While the specific details of the YDIH vary from publication to publication, the general premise is that at ~ 12.9 ka North America and other continents were subjected to some sort of extraterrestrial ‘event’ (either supernova shockwave; meteoritic, cometary, or very low-density object - impact(s); bolide airburst(s); or some combination thereof).
This is false. It is clear from its inception in Firestone et al. (2007) that the YDIH posits
“… that one or more large, low-density ET objects exploded over northern North America … The evidence is more consistent with an impactor that was carbon-rich, nickel–iron-poor, and therefore, most likely a comet.”
The updated outline of the hypothesis in Wolbach et al. (2018a), reiterated several times since (e.g. Pino et al. 2019, Moore et al. 2023), makes it clear that the preferred impact scenario involves a previously fragmented comet (see our Section 3).
However, it is natural in science to adjust a hypothesis to better fit the observed evidence. Thus, for a while, the Hiawatha crater did provide an alternative to the ‘fragmented comet’ hypothesis, with several publications exploring how it may have been a candidate for the YDIH (Moore et al. 2019; Beech et al. 2020). While the crater initially appeared to be very young based on its morphology, remanent geothermal energy, impact-modified carbon from Pliocene/Pleistocene conifers, and more, recent evidence from the site has cast considerable doubt on such links (Kenny et al. 2022; Hyde et al. 2023). A supernova shockwave has never taken the place of an ET impact in the YDIH.
HEA: The term ‘impact’ in “YDIH” represents all these possible cosmic events.
It is true that several ET impact scenarios involving low-density objects have been proposed over the history of the YDIH (see our Section 3). But to claim that the modern YDIH, as defined at the time HEA’s manuscript was written, includes all the cosmic events listed above is patently false. To make their claim, HEA must lump together unspecified claims from a wide range of sources as though they carry equal weight with specific claims made by core members of the original Firestone et al. (2007) team. This is unreasonable and a corruption of the scientific method.
HEA: That event supposedly caused climate changes that define the onset of the YD stadial (see Firestone and Topping, 2001; Firestone et al., 2006, 2007; Kennett et al., 2008a, 2009a; Bunch et al., 2012; Israde-Alcántara et al., 2012; LeCompte et al., 2012; Wittke et al., 2013a; Moore et al., 2017; Kennett et al., 2018; LeCompte et al., 2018; Sweatman 2021; Powell, 2020, 2022). More significantly, YDIH proponents claim that the proposed impact at the beginning of the Younger Dryas (i.e., the lower “Younger Dryas Boundary [YDB]”) “triggered an ‘impact winter’ and the subsequent Younger Dryas (YD) climate episode, biomass burning, late Pleistocene megafaunal extinctions, and human cultural shifts and population declines” (Wolbach et al., 2018a, abstract), among other claims.
Correct. Secondary effects of the YD impact, including the YD cooling anomaly, megafaunal extinctions, and effects on human populations and cultures have been important components of the hypothesis since its inception, and continue to be today. However, as with the Hiawatha crater, the YDIH does not live or die by the truth of any one of these secondary processes.
HEA: A comprehensive and self-consistent statement that describes the YDIH, clarifies confusing/contradictory data, arguments, and interpretations does not exist.
This is false. Wolbach et al. (2018a) provides a consistent and comprehensive update statement of the YDIH (see the Introduction) and was discussed in Holliday et al. (2020).
HEA: This paper is an in-depth critical review of the data and interpretations used to both promote the YDIH and counter critics of the YDIH, including recent summary reviews of the hypothesis (Sweatman, 2021; Powell, 2020, 2022).
Use of the word “promote” is pejorative. The recent reviews of Sweatman (2021) and Powell (2022) are peer-reviewed analyses that focus specifically on the physical evidence, unlike HEA which includes derision and entire sections of ad hominem attacks (see later).
HEA: In the following discussion, we make liberal use of direct quotes to clarify communication disconnects that seem to characterize the debate and to better make our points. We repeat some of the critiques from previous papers. The reason is obvious, as is apparent throughout this paper. The vast majority of critiques and contradictory data have never been directly addressed by YDIH proponents.
Wolbach et al. (2020) responded directly to Holliday et al. (2020) and Kennett et al. (2015b) responded directly to Holliday et al. (2015) and Boslough et al. (2015). Other critiques of the YDIH are so obviously flawed that they should be withdrawn (see later sections) or are also Gish gallops (see our Introduction for an explanation of this term), like HEA, and were therefore ignored. We respond to HEA now partly to correct their scientific misconceptions and partly to challenge their derision and thinly veiled accusations of misconduct.
HEA: Critiques of the YDIH were published by researchers in a broad array of fields regarding reproducibility of results, extinctions, Clovis archaeology, stratigraphy, dating methods, YDC climate change, mineralogy, geochemistry, statistical probability, and impact physics, among other topics.
HEA: Proponents of the YDIH have argued that such critiques have been addressed, but either provide no citations or when provided, those citations do not adequately address the critiques (see Table 1). For example, Kennett et al. (2015b, p E6723) assert that criticisms that purported YDIH “impact proxies” also occur in multiple horizons outside the YDB were “refuted in detail” (citing Kennett et al., 2015a; Bunch et al., 2012; LeCompte et al., 2012; Wittke et al., 2013b). Similarly, Sweatman (2021, p 14) falsely asserts that rebuttals to Wolbach et al. (2018a, b) “were already addressed” but provides no references regarding those claims. Holliday et al. (2020, table 2) list eleven major claims based around the YDIH that are either partially or completely unaddressed in the YDIH literature. Table 1 summarizes the limited rebuttals to critics of the YDIH. Wolbach et al. (2020) provide the only lengthy attempt to rebut criticisms, but most of those rebuttals either repeat claims regarding the YDIH previously dismissed or miss the key points raised by critics (Table 1). This review demonstrates that the YDIH is untenable in the light of research since its initial conception.
The evidence and arguments used by HEA and by earlier critiques are flawed and are addressed here, line-by-line, at least within the chosen sections of HEA. If the YDIH is untenable as a hypothesis, then how can evidence from 58 YDB sites that corroborates it contained in scores of peer-reviewed articles be explained?
[Table 1 is omitted for brevity]
HEA: The YDIH has a long, checkered history that is not rooted in science (see Daulton et al., 2017a, p. 7).
The YDIH began as a scientific proposal in Firestone et al. (2007). The physical evidence supporting the YDIH is contained only in peer-reviewed articles since 2007. Earlier non-refereed publications aimed at the general public might be of interest to historians, but they are irrelevant to the scientific debate which has taken place in scientific peer-reviewed journals since 2007.
Ultimately, the truth of the YDIH will be settled solely by the physical evidence described in peer reviewed publications since 2007. All other forms of evidence and argument are irrelevant. HEA’s interest in other texts indicates they are not motivated solely by the science, leading to a corruption of the scientific method.
HEA: One of the earliest versions of the hypothesis is the speculative book by Donnelly (1883), which claims a comet struck North America forming the Great Lakes. As the story goes, the aftermath devastated human (in particular) and other faunal populations and plunged the climate into a period of extreme cold (or a return to glacial conditions).
Clearly, this idea is similar, but it is not the same as the YDIH which was only defined scientifically in 2007. But why stop at Donnelly? Using HEA’s logic, we could extend the association back further and cite Whiston’s comet, or the Bible’s great flood, or the Enuma Elis’s Bull of Heaven, or the long-tailed heavenly climbing star of the Ojibwe as the ultimate source for Firestone et al. (2007). The reality is that an ancient cosmic catastrophe is pervasive in folklore, religion and culture worldwide. For example, James and van der Sluijs (2016) analyze the Phaethon myth and similar stories from around the world and conclude;
“The classical myth as it stands has demonstrable origins in Near Eastern precursors. The much wider problem, raised almost a century ago by Frazer, concerns the extraordinary matches found outside the Old World. Here only a wider approach towards the astronomical possibilities—beyond a simple meteorite fall—can provide a plausible explanation for both the similarities and the differences between the global traditions. … Such an explanation of the global parallels is preferable to the idea of (unexplained) diffusion.”
Clearly, the YDIH could provide an explanation. Nevertheless, while there may be some truth in these stories, they are not useful from a scientific perspective for assessing the YDIH. As already stated, only the physical evidence published in peer-reviewed journals since 2007 is relevant. Attempts to connect the hypothesis backwards in time to earlier stories is a well-known rhetorical device known as a “strawman” argument and a corruption of the scientific method. Indeed, using HEA’s strategy, almost any major modern scientific theory, including biological evolution, can be disputed by criticizing their earlier speculative precedents. In fact, it is a feature of science that progress is often made through the critical evaluation and testing of earlier speculative ideas that, although eventually found to be wrong, were useful in prompting further research. Thus, HEA’s criticism is anti-science.
HEA: This idea was resurrected by R. Firestone in a series of popular magazine comments and a popular-press book. Firestone and Topping (2001) embraced and combined earlier, long-rejected ideas predating modern understanding of impact craters to argue that the Carolina Bays are Late Pleistocene impact structures (Melton and Schriever, 1933; Sass 1944; Eyton and Parkhurst 1975) and that a supernova irradiated the Earth in the Late Quaternary (Brakenridge, 1981). Subsequently, Firestone’s focus shifted from a supernova (Firestone and Topping, 2001; Firestone 2002) to Donnelly’s (1883) comet that created the Great Lakes. This shifted focus is described in the book The Cycle of Cosmic Catastrophes: How a Stone-Age Comet Changed the Course of World Culture (Firestone et al., 2006) and a journal article (Firestone et al., 2007) (see also Section 7).
Firestone et al.’s (2006) book is a speculative work aimed at the general public and not at the scientific community. We reiterate that the initial scientific case for the YDIH was presented by Firestone et al. (2007). Works that predate Firestone et al. (2007) may be of interest to historians, but they are clearly of no relevance to the scientific debate which has occurred in peer-reviewed journals since 2007. HEA’s continued attempts to extend the scientific debate backwards before 2007 is, again, a strawman fallacy and a corruption of the scientific method. As stated above, HEA’s criticism is not scientific since most major modern scientific theories developed through a series of earlier speculative ideas. Essentially, HEA’s criticism leaves no room for speculation and error which are both essential components of the scientific method. The whole point of the scientific method is that it is self-correcting.
HEA: As for the supernova, it was then claimed to have perturbed the orbit of a solar comet (Firestone et al., 2006) or ejected an exosolar comet (Firestone et al., 2006, Firestone 2009a, b) that struck the Earth.
HEA: Firestone et al. (2006, 2007) were the first publications to gain wide attention, in part due to an AGU symposium in 2007 that drew considerable attention from the news media.
This observation is irrelevant to the scientific debate as it contains no arguments based on physical data.
HEA: The book is based on fanciful speculation and demonstrates a remarkable lack of understanding of the archaeological and stratigraphic data discussed. It contains many examples of misleading or blatantly untrue statements (noted throughout this review) and was described by Morrison (2010) as “pseudoscience.”
This is false, another strawman fallacy, derisory and irrelevant. Morrison’s opinion is published in a popular science magazine and is not peer-reviewed by independent scientists. Nor is the book it is based on relevant to the scientific debate.
HEA: The 2006 book and the other papers were not an auspicious prelude to the 2007 paper by Firestone et al.
This is yet another strawman fallacy. Since these earlier publications are irrelevant to the scientific debate in peer-reviewed journals since 2007, HEA’s opinion of them is irrelevant.
HEA: Further, that 2007 paper has problems including: a) poor-to-nonexistent numerical age control for most sites (see Section 5.3); b) no data on identification of nanodiamonds, polycyclic aromatic hydrocarbon (PAH) molecules (see Section 9.3), and fullerenes with extraterrestrial (ET) helium (see Section 13.2); c) highly speculative interpretations of the origins of magnetic spherules (see Section 10) and carbon spherules (see Section 12.4); and d) failure to publish a table of the measured concentrations of their proposed markers that they used to generate ambiguous graphs (see Section 13.6). That publication, as well as many subsequent papers by the YDIH proponents, contains many significant and obvious misstatements of fact, circular reasoning, and problematic age control, all reviewed here.
We agree that Firestone et al. (2007) was not the last word in the YDIH debate. This is why many articles since its publication have provided more details about nanodiamonds, microspherules and other forms of impact debris in the YDB. To refute the YDIH, HEA should focus their attention on the current state-of-the-art of research in these articles. Moreover, the claimed “misstatements of fact’ in these later papers are misunderstandings on HEA’s own behalf. If their accusations were true, why were they not caught by hundreds of peer reviewers who approved publication of scores of articles authored by dozens of scientists on the YDIH over the last 17 years?
HEA: Misunderstanding or misstating stratigraphic and archaeological records is a common theme in support of the YDIH, as documented in this review and elsewhere. Claiming evidence where none exists and providing misleading citations may be accidental, but when conducted repeatedly, it becomes negligent and undermines scientific advancement as well as the credibility of science itself.
It is human to err and errors are common in scientific papers. Indeed, we identify many egregious errors in HEA in this and later sections. However, we strongly dispute this baseless implied suggestion of scientific misconduct. Instead, these are mainly misunderstandings on behalf of HEA, as will become apparent.
HEA: Also culpable is the failure of the peer review process to prevent such errors of fact from entering the literature. The Proceedings of the National Academy of Sciences “contributed review” system for National Academy members (e.g., Aldous, 2014), as in the case of Firestone et al. (2007) and Kennett et al. (2009a), is at least partially responsible. The “pal reviews” (as some refer to them) were significantly curtailed in 2010, in part due to the YDIH controversy.
Once again, HEA are implying misconduct which we strenuously dispute. As already stated, the truth of the YDIH rests on the physical evidence alone. The argument provided by HEA here is derisory and much of the evidence and arguments HEA use to support their case are fundamentally flawed.
Moreover, no evidence is provided to support the claim that the “pal reviews” were “significantly curtailed in 2010, in part due to the YDIH controversy.”
HEA: We begin our review of the YDIH by examining its foundation; specifically, we probe the enigmatic questions the YDIH attempts to answer, and the assumptions behind those questions. To place the archeological, paleontological, and paleoclimatic questions the YDIH attempts to answer into proper context the “Younger Dryas” is defined in Section 2. In Section 3, the assumptions that underpin the foundational questions of the YDIH are examined in detail, showing that several are flawed or fundamentally false, any one of which would reject the overall hypothesis.
The underlying assumptions and propositions of the YDIH include:
HEA: a) The environmental changes at the beginning of the YDC are synchronous around the world. This assumption is probably true, and is supported by high-resolution, independently dated speleothem and lake records (Section 3.3), but synchroneity is not unique to the YDIH.
The phrase “synchroneity is not unique to the YDIH” is unclear. Perhaps HEA refer to the dating of YDB sites? While we would naturally prefer the dates of all YDB sites to be both consistent and precise, we must instead interpret the evidence as it is found rather than as we would like it to be. In other words, we can have no expectation a priori that the dates of YDB sites will always be precise, but we can have an expectation that they are not inconsistent with synchroneity. HEA’s view that the dating of all YDB sites must be both consistent and precise is an unrealistic demand. So far, no YDB site has been found to be obviously inconsistent with the proposed date of the YD impact.
HEA: b) The direct effects of the hypothesized impact were synchronous around the globe and date precisely to the YDB. This is clearly contradicted in archaeological, paleontological, and paleoenvironmental records (Sections 3, 5, 13.1, 13.7).
Again, it is not entirely clear as to what this statement refers. If HEA are referring to the onset of the Younger Dryas cooling, then evidence from the GISP2 ice core shows that a clear platinum signal is synchronous with the onset of rapid cooling in central Greenland to within experimental uncertainty. If they refer to the demise of the Clovis culture then we respond that evidence relating to the demise of Clovis is in good accord with the YDIH and, moreover, no studies have yet found any Clovis artifacts above the YDB (see below and the next section). If they refer to the end-Pleistocene megafaunal extinctions, then we respond that independent studies have shown that the extirpation/extinction of many megafaunal species are strongly correlated with the date of the YD impact (see our Section 4 above).
This issue is really concerned with radiocarbon dating of these “archaeological, paleontological, and paleoenvironmental records”. As we show in the next section, HEA misinterpret the uncertainty in such data. In fact, to properly test the YDIH, as already emphasized by Sweatman (2021) and in our Section 4 above, any study of such “archaeological, paleontological, and paleoenvironmental records” should always compare them with the YDB. Any study that does not can automatically be questioned.
HEA: c) The direct and indirect effects of the hypothesized impact were consistent in sign, pattern and magnitude with the “Impact Winter” scenario (or with nuclear winter or exceptional volcanism scenarios). This is contradicted by the spatial pattern of YD climates (Section 3.3).
The YDIH allows for both an impact winter scenario and massive changes in oceanic circulation (AMOC) to impact climate. This is stated explicitly in Firestone et al. (2007) and reiterated in Wolbach et al. (2018a) (see our Section 4). The interaction between any putative impact winter and an altered AMOC on the climate remains to be investigated.
Moreover, it is only HEA’s opinion that these effects can be distinguished using the low-resolution data they consider in their Section 3.3. We recommend that detailed sub-annual paleoclimate records should be used instead because the proposed impact winter is described as lasting only a matter of weeks or months by Wolbach et al. (2018a, b).
HEA: d) The YD (and its accompanying climate reversals) was a unique episode during the Quaternary and requires a special explanation. This is contradicted by numerous long terrestrial, marine and ice-core records, which demonstrate that hundreds of such episodes occurred during the Quaternary (Section 3.3).
The mere existence of numerous climate episodes during the Quaternary says nothing about how they occur. Even if they are generally consistent with the AMOC-halting hypothesis, there could be some that are not or some for which the AMOC-halting mechanism is triggered by a catastrophic event. The mechanism for each episode should be investigated separately. There could be many different kinds of trigger, or just a few. A cosmic impact trigger might be common or limited to just the YD onset. The YDIH makes no claims about these other episodes. Indeed, the assumption that the trigger for any such episode cannot be related to cosmic impacts is patently unsound and no evidence is presented by opponents of the YDIH to support this view.
HEA: e) Clovis Paleoindians disappeared immediately after the impact. The ‘disappearance’ of Clovis was no more than an instance of cultural change, technological change and/or a change in settlement strategy (Section 3.1).
No YDIH publications have claimed that the Clovis people all disappeared immediately on the date of the impact event. Moreover, HEA’s assertion that the decline of Clovis involved only “cultural change, technological change and/or a change in settlement strategy” is overconfident. As with many other issues in this area, interpretation of the evidence depends strongly on radiocarbon dating of archaeological sites. We emphasize again that any study that does not compare the “archaeological, paleontological, and paleoenvironmental records” to the YDB can automatically be questioned. Thus, this argument is an extension of b).
In fact, Haynes (2008) states that;
“… no post-Clovis Paleoindian artifacts have ever been found in situ stratigraphically below [the YD black mat]”,
of which the lower boundary often marks the position of the YDB. He further states that;
“The YD black mat covers the Clovis age landscape on which the last skeletal remnants of Rancholabrean megafauna occur” and although the “ages of the youngest Clovis sites overlap with those of the oldest Folsom sites…the stratigraphic separation is clear…The megafaunal extinction and the Clovis-Folsom transition appear to have occurred in <100 years, perhaps much ess.”
Anderson et al. (2011) also explicitly state that there was a population decline/reorganization related to the impact event, at the same time that the majority of the East Coast chert quarries were abandoned. This view is aligned with the most recent radiocarbon evidence which is interpreted by Waters et al. (2020) to indicate;
“Clovis — the technology — abruptly ends at ~12,750 cal yr B.P. and coincides temporally with the beginning of the Younger Dryas cooling event and paleontologically, with the extinction of Mammut, Mammuthus, and Cuvieronius. Archaeologically, Clovis terminates immediately before the emergence of Folsom technology on the Plains (27, 46) and the eastern Fluted Point Tradition in the eastern United States (47). In contrast, the production of stemmed points in the western United States continues after Clovis ends (48).”
HEA: f) Megafauna extinctions began immediately following the impact (although extinctions are also claimed by some YDIH proponents to have occurred from multiple impacts over tens-of thousands of years). Many genera have last appearance ages that predate the YDC by millennia, and others survived to the end of the YDC or into the Holocene (Section 3.2).
The YDIH concerns only those extinctions clustered around the YD onset, sometimes called ‘Rancholabrean’. Haynes (2008) states that;
“No skeletal remains of horse, camel, mammoth, mastodon, dire wolf, American lion, short-faced bear, sloth, tapir, etc., or Clovis artifacts have ever been found in situ within the YD age black mat.”
This fact is consistent with the YDIH.
Moreover, as there is good independent evidence of a cluster of extinctions strongly correlated with the YD onset (e.g. Stewart et al. 2021, O’Keefe et al. 2023), there is naturally a good case they were triggered by the YD impact. Even reports that claim to contradict the possibility of megafaunal extinctions linked to the YD impact, such as Gill et al. (2009), support it when properly analyzed (see our Section 4 above).
No claims are made about other extinctions by the YDIH, but it is an open question as to whether cosmic impacts played a role more generally (e.g. see Napier 2010). HEA’s assumption that cosmic impacts cannot have played a role in any of these extinction events is patently unsound and HEA provide no evidence to support this view. In the case of the K-Pg extinction, the disappearance of species prior to the impact is argued to be an artifact of the Signor-Lipps effect.
HEA: g) The demise of Clovis technology, and megafauna extinctions were unique, discretely dated events and require special explanation. These are baseless interpretations or assertions that contradict extensive data sets (Sections 2, 3, 5, 13.1, 13.7).
No YDIH publication has claimed that these were simultaneous “discretely dated events.” Rather, the YDIH recognizes that extinction events may play out over centuries to millennia, especially because small groups of megafauna can survive in refugia. Even so, the apparently synchronous timing of YD climate change, a cluster of Rancholabrean megafaunal extinctions and cultural changes (including Clovis) with widely dispersed impact proxies at the YDB suggests that the driving force of the YD climate change, extinctions and the demise of the Clovis culture was the impact event ~12,800 years ago. Again, this argument is really about how the YDB and archaeological and paleontological records are dated using radiocarbon, and whether they are compared directly with the YDB, and is therefore an extension of b) and e).
HEA: h) The sampling for data from sections spanning only hundreds or a few thousand years is sufficient to categorize an event as unique and unprecedented within many millennia. Long, well-dated sections with records of uninterrupted deposition must be subjected to discrete, continuous sampling and analysis to demonstrate the uniqueness of any claimed event of suite of purported impact indicators (Section 4). No such sections and data sets have been reported.
This presumably refers to the geochemical impact proxies found at > 50 YDB sites and is an important point. The dating and synchroneity of the YDB at these sites is dealt with in detail in the next section.
HEA: i) The beginning of the YDC must be determined using terrestrial age control. The YDC is defined as a component of the geologic time scale and its lower and upper boundaries are defined by Greenland ice-cores, supplemented by speleothem and other annual-resolution records (Section 5.1).
Proponents of the YDIH are working to a different model where the YD onset was triggered by an ET impact. According to this model, the GISP2 platinum spike defines the onset of the YD in Greenland ice cores and coincides with a significant reduction in temperature in central Greenland. This platinum abundance was airborne for around 20 years and therefore should be global, as remarked by Petaev et al. (2013). A platinum anomaly has been detected at many YDB sites (Moore et al., 2017) on several continents coincident with other indicators of an ET impact, such as microspherules and nanodiamonds. Thus, the YD onset is correlated between Greenland ice cores and terrestrial records via the YDB and the platinum signal.
While the suggested impact-induced winter would affect the whole globe synchronously to varying extents, the YD climate change which followed likely also involved disruption of major ocean currents (the AMOC hypothesis) and thus its effect might not be synchronous across the whole globe. Note that seeking correlations between ice cores and other records is fraught with uncertainty. For example, typical speleothem records have a resolution of > 10 years while the GICC05 ice core chronology has a maximum counting error of 140 years. The duration of any impact winter, on the other hand, is suggested by Wolbach et al. (2018a, 2018b) to be < 1 year.
HEA: j) Numerical age control is accurate and precise at most sites with impact indicators and statistically conforms to a singular geologic event. Most sites lack directly dated samples from within their purported YDB layers and on adjacent layers, and even among those that have such samples, their dates vary between sites and many dates lack precision (Section 5). Age-depth models provide only an estimated age, typically with large statistical errors.
Currently, impact-related debris in the YDB at 17 sites on four continents is dated to within a 1,000-year period around the expected date of the YD impact (see our Section 6 later). The possibility this debris resulted from anything other than a single ET impact event or a highly correlated series of events within a short time span is negligible.
YDIH opponents claim that individual samples from within the YDB layer should be used for “age control”, and that these must be consistent and precise. This is an unreasonable demand considering the uncertainty inherent in radiocarbon dating. Again, this is an argument about dating, like b), e) and g). See also the next section. HEA consistently fail to recognize, as shown in our Section 4, that the use of individual radiocarbon measurements from any site is unreliable because the intrinsic radiocarbon measurement uncertainty is not a reliable estimator for the true sample age uncertainty. Instead, a suite of measurements from around the YDB will provide a much better estimate of the sample age uncertainty and can be used to create age-depth models. This is widely acknowledged as best practice. Moreover, any study that does not compare the “archaeological, paleontological, and paleoenvironmental records” to the YDB can automatically be questioned.
HEA: k) So-called “black mat” deposits and the Usselo/Finow soils are unique, date to the YDB (or YDC, depending on the version of the YDIH), and are a consequence of the impact. These organic-rich soils and sediments comprise a major source of confusion and contradictions surrounding YDIH. They are not linked to the YDB, and few examples are unique to the YDC (Sections 5.6, 6).
HEA’s assertion that the Usselo/Finow charcoal-rich horizons “are not linked to the YDB” is overconfident and runs contrary to evidence which shows that geochemical indicators of an ET impact are found within these layers at several sites. This evidence is confirmed by multiple independent research groups (see, for example, van Hoesel et al. (2015)). HEA provide no evidence that the Usselo/Finow charcoal-rich horizon layers cannot be related to the YD impact, and the “confusions and contradictions” mentioned are word-play by YDIH opponents. Again, this is an argument about radiocarbon dating, like b), e), g) and j) and is described in more detail in the next section.
HEA: l) There is a simple YDB impact scenario consistent with known physics and all the purported evidence. Various (often conflicting and disjointed) impact scenarios have been proposed and are necessary to explain the wide range of physical sediment constituents offered in support of an “impact event”, i.e., supernova event, surface impact(s), and/or aerial bolide(s) (Section 7). The YDIH is a collection of different variant hypotheses (and impact scenarios) that attempt to use the same purported set of evidence with unavoidable conflicts and contradictions.
As already explained in our Section 3, this is false. The update in Wolbach et al. (2018a) is a concise and coherent description of the YD impact event.
Once again, HEA use a strawman fallacy to link the YDIH to publications earlier than Firestone et al. (2007) and other non-peer-reviewed articles, but this is a corruption of the scientific method.
HEA: A broad array of physical evidence is claimed by YDIH proponents to support the various impact scenarios. Proponents of the YDIH make several assumptions in their interpretation of the physical evidence and these include:
HEA: m) Craters that date to the YDB may or may not exist regardless of the purported evidence (to the contrary see Sections 7, 8, 13.1). Craters provide the strongest evidence of an impact and those dating to ~12.9 ka should be well preserved, but none are known (Section 8).
Firestone et al. (2007) did not predict or require the presence of a YD impact crater. Instead, they wrote,
“We propose that one or more large, low-density ET objects exploded over northern North America, partially destabilizing the Laurentide Ice Sheet and triggering YD cooling.”
Neither is an impact crater required by the updated YDIH scenario in Wolbach et al. (2018a, b) or in Moore et al. (2023). Thus, impact craters are not yet a requirement of the YDIH, a fact already highlighted by Sweatman (2021), and therefore HEA’s argument is another “strawman” fallacy. Nor are craters a requirement for many other known fields of impact glass or spherules (for example, see Osinski et al., 2008). Those other events are widely accepted to be caused by impacts, and yet, no craters have been found. But, obviously, discovery of a YDB-age crater will tend to confirm the YDIH. This position has been consistent throughout the debate.
However, large amounts of impact microspherules with mainly terrestrial composition within a narrow timeframe on several continents does require some kind of surface alteration (from airbursts) or cratering (from ground impacts). But craters can be elusive, e.g. if they are under water, and surface scarring generated by airbursts can easily be missed today, and so their apparent absence to date does not negate the YDIH, much like the initial absence of a crater did not negate the Chicxulub impact.
HEA: n) The charcoal record of fire has been interpreted correctly and shows “the entire continent was on fire” (J. Kennett in Pringle 2007). The data on wildfires cannot be used to unambiguously indicate the extent, type, intensity or temperatures of fire (Section 9). The global charcoal record has been subject to various misapprehensions and misinterpretations (Section 9.1) and when reanalyzed by YIDH proponents’ yields results similar to that in the literature. Multiple peaks in charcoal abundance are documented through late Quaternary sections, but none have been shown to be uniquely associated with an impact.
We agree with HEA that it is not possible to show that a charcoal abundance is uniquely associated with the YD impact since charcoal is common in soils and usually related to non-impact wildfires. So, their complaint here is unreasonable. Nevertheless, we can expect a cosmic impact to generate significant wildfires, and therefore a charcoal abundance, or an abundance of other forms of carbon/soot, are expected around the YD onset. In fact, peaks in the abundance of charcoal and ammonium ions (a proxy for wildfires) near the YD onset have been found on several continents and Greenland (see o) below). Thus, the wildfire evidence corroborates the YDIH.
HEA: o) The ice-core record of fire was interpreted correctly and shows a big peak in fire at the YDB. YDIH proponents have badly misinterpreted the ice-core record (Section 9.2). The ice core and charcoal records agree that the YDC (and the YDB in particular) was a time of low incidence of fire (Section 9).
Both terrestrial records of charcoal and Greenland ice core records of ammonium ions (a proxy for wildfires) show clear peaks in abundance centered around the YD onset within uncertainty bounds. See, for example, Figure 1C in Marlon et al. (2009), Figures 3C, 3D, 4C and 4E in Wolbach et al. (2018a), and Figures 4b and 4c in Fischer et al. (2015).
HEA: p) Spherules and microspherules are unambiguous indicators of an extraterrestrial impact and/or impact-generated wildfire. Microspherules can have various origins other than impact and cannot be used as impact indicators unless they are shown to be of meteoritic origin, which is not the case for most purported YDB microspherules (Section 10). The YDB carbon spherules are not impact-generated wildfire products but rather are fungal sclerotia that are ubiquitous in sediments (Sections 9.3, 12.4).
HEA largely ignore the most detailed and compelling microspherule evidence found in, for example, Bunch et al. (2012), LeCompte et al. (2012), Israde-Alcántara et al. (2012), Wittke et al. (2013), Andronikov et al. (2016), Pino et al. (2019), and Moore et al. (2020). Mostly these microspherules have a terrestrial composition, consistent with being impact-melted target rocks, but meteoric components are commonly present. These studies contradict HEA’s conclusions, yet they offer no alternate explanation at all for the widespread abundance of microspherules in the YDB together with an assembly of other impact proxies such as nanodiamonds and platinum. Instead, HEA indefensibly argue that this assembly of apparently synchronous impact proxies across four continents is coincidental.
French and Koeberl (2010) indicate that ET impacts can be detected on the basis of microspherule evidence alone, and even list criteria by which this can be achieved (see Section 7.3). The microspherules recovered from the YDB at Abu Hureyra appear to satisfy these criteria, essentially confirming an ET impact there. This view is supported by the presence of other impact proxies, such as lechatelierite, excess platinum and nanodiamonds, in the YDB at that site.
A similar assemblage of impact proxies is found within the YDB at many other sites, thus indicating ET impacts at those sites too. The carbon microspherules containing nanodiamonds very likely result from the impact while others may derive from other wildfires.
HEA: q) Platinum-group element measurements of YDB sediments and ice provide support for an impact (to the contrary see Section 11). Platinum anomalies can arise from terrestrial sources and those reported by YDIH proponents are not uniquely associated with the YDB (Section 11).
It is true that platinum anomalies can also arise from volcanism, but platinum co-occurs with zircons and “first quartz,” e.g., deviatoric elastic strains in quartz crystals erupted from volcanoes (microscopic, bipyramidal, euhedral quartz crystals). These proxies are absent in Younger Dryas Impact site strata. Conversely, well-established proxies for cosmic impact events (e.g., nanodiamonds, lonsdaleite, cosmic impact microspherules, iridium, etc. reported at YDB sites) are absent on catastrophic volcanic impact sites (Tankersley et al. 2011, 2015, 2016, 2018a, 2018b, 2020). Given the platinum is coincident with other impact proxies in the YDB, it is almost certainly extra-terrestrial and is therefore very likely the global signal expected after discovery of the GISP2 platinum spike by Petaev et al. (2013).
HEA: r) Techniques and methods used to measure nanodiamond abundances are correct and accurate, nanodiamond identification is also correct, and nanodiamonds are reliable impact indicators. In most cases nanodiamond identification is suspect. All measurements of nanodiamond concentration in sediments/ice is scientifically meaningless, and in several cases irreproducible by YDIH proponents (Section 12).
Abundance peaks of nanodiamonds are not scientifically meaningless, especially if they are synchronous with other impact proxies on at least four continents. Nanodiamond identification is not suspect in general, although there are issues related to identifying the precise nanocrystalline form of diamond and the precise concentration. This is a rapidly-evolving research area in itself. Nanodiamonds at the YDB have been identified by multiple independent research groups, including opponents of the YDIH. This is the gold standard in science.
6. Rebuttal of HEA’s Section 5: Inadequate Dating and Stratigraphic Context
It is apparent from the previous sections that a major area of disagreement with HEA concerns the interpretation of radiocarbon dating evidence relating to the “archaeological, paleontological, and paleoenvironmental records” and the YDB. We expect that many of HEA’s misunderstandings of the YDIH can be traced to this issue. Therefore, in this section we focus on HEA’s Sections 5.3-5.8 where this issue is discussed in detail. We respond line-by-line to HEA’s text below; our comments are indented in italics.
6.1. HEA’s Section 5.3. Deficient Dating of YDIH Sites
HEA: Dating fundamentals in the context of the YDIH debate are summarized by Holliday et al. (2014, p 519) but bear repeating here from Holliday et al. (2020, p. 70) given how crucial the issue is on both sides of the debate: “Reliable and precise numerical age control for stratigraphic sections and associated samples is a key component of the YDIH debate. Proponents recommend ‘very high chronological resolution to test the hypothesis’ [Kennett et al., 2008a, p. 2531].
HEA are suggesting here that there is too much uncertainty in some of the claimed YDB sites for them to be useful. Therefore, evidence from those sites should be rejected. This is incorrect for reasons that will be explained later. Of course, we must deal with the evidence as it is found, not the evidence we would like. In fact, opponents of the YDIH need to show that dating of purported YDB sites is obviously mutually inconsistent. To achieve this, they need to show with high confidence that the difference in the age of the YDB at a given site and the suggested age of the YD impact is far larger than the uncertainty in any of these age estimates. For YDIH proponents the converse is true. Since there are on the order of 50 YDB sites to consider, a difference of 4 or 5 times the standard error would be worrying. Much more than this would be fatal. However, remember that age estimates based on single radiocarbon measurements are unreliable and should be considered with caution.
The most detailed study of YDB site dating is by Kennett et al. (2015a), which established using Bayesian modeling a date for the YD impact of 10,835 BCE ± 50 years (2-sigma or 95 % confidence). However, the radiocarbon calibration curve has been updated since 2015. According to Cheng et al. (2020), using the latest radiocarbon calibration curve this date is shifted to 10,875 BCE ± 50 years. Mostly, the research reviewed below uses the older calibration curve.
HEA provide no evidence that the age of the YDB at any site is inconsistent with this date.
HEA: Furthermore, they argue that ‘only’ radiocarbon dates with precisions of ‘<100 years, and preferably <60 years’ [apparently meaning 14C years] should be used for dating the YDB layer and complain that many dates employed by others have ‘precisions from 200 years to >2,000 years’ [Kennett et al., 2008b, p. E107].
Clearly, more precise dates are desirable. But there should not be any cutoff in the acceptable precision of a YDB site. Less precise dates for the YDB are still useful, as we shall see.
HEA: They also propose that the only valid dates are those processed with ‘modern techniques [e.g., XAD… or ultrafiltration].’ Given that the debate is about whether some sort of extraterrestrial event created an environmental catastrophe at a precise moment in geologic time, we agree that accurate and high-precision dating is essential for testing the hypothesis.”
There is no initiating quote mark here, so it is not clear if this is a quote or not. In fact, as stated several times already, “accurate and high-precision dating” is not essential. Even much less precise dates are still useful for testing the hypothesis, as we shall see.
HEA: Kennett et al. (2015a, p 4344) wrote, “In a test of synchroneity, it is ideal to have numerous, highly accurate, and precise dates to develop robust chronological models.” Unfortunately, no dates used to support the YDIH meet these requirements, and very few sections or samples are so accurately or precisely dated (Table 4).
Of course, numerous precise dates are ideal. But we must deal with the evidence as it is found, not as we wish it to be. Moreover, the phrase “Unfortunately, no dates used to support the YDIH meet these requirements” is a distortion because no “requirement” is stated in the quote provided. Only a preference is stated. As already stated many times, less precise dates are still useful.
HEA: LeCompte et al. (2018, p156) complain that YDIH critics “do not use rigorous dating methods…” A more critical issue is that the YDIH proponents do not meet this standard. Indeed, “precisions from 200 years to >2,000 years” (Kennett et al., 2008b, p. E107) characterize the results presented by Kennett et al. (2015a).
The word “complain” is a pejorative and not suitable for a scientific publication. The issue of less precise dating of some YDB sites has already been dealt with many times.
HEA: Besides misunderstanding and mischaracterizing Clovis archaeology and extinctions (Sections 1.0, 3.1, 3.2, and 5.7), …
YDIH proponents do not share HEA’s overconfidently stated opinions on these matters. The disappearance of the Clovis technocomplex from the archaeological record appears to be in accordance with the YDIH (see HEA’s Section 5.7 below). Moreover, there is good evidence for a cluster of megafaunal extinctions close to the YD onset (see our Section 4).
HEA: … the basic dating for the hypothesis proposed by Firestone et al. (2007) was fundamentally flawed at the outset. More broadly, radiocarbon dating has been a long-standing conundrum for the YDIH (see Firestone and Topping, 2001; Southon and Taylor, 2002; Firestone 2009a, b, 2014; Gillespie 2009; Melott et al., 2015). Firestone et al. (2007, p 16017) state that “Ten Clovis and equivalent-age sites were selected because of their long-established archeological and paleontological significance, and, hence, most are well documented and dated by previous researchers.” This is not the case, as thoroughly discussed and documented by Meltzer et al. (2014) and summarized in Table 4. At best, only three of the sites (Blackwater Draw, Murray Springs, and possibly Daisey Cave) have reasonable age control and four have very poor to no age control (Chobot, Gainey, Morley, and Wally’s Beach).
Yet again, by “age control” HEA refer to precise radiocarbon dates for the YDB. But as already stated many times, we must deal with the evidence that is found, not the evidence we would like. In fact, even much less precisely dated YDB sites are still useful.
The arguments and evidence in Meltzer et al. (2014) are considered later; we will show that their methodology is flawed and thus their conclusions are not supported by their evidence. This fact had already been highlighted by Sweatman (2021) and others.
HEA: Firestone et al. (2007) also allude to stratigraphic correlation with and sampling of their purported YDB in 15 Carolina Bays but provide no stratigraphic nor geochronologic data (Table 4).
The main dating evidence is in Kennett et al. (2015a) which supersedes Firestone et al. (2007).
HEA: Subsequent investigations of the YDIH produced additional attempts at age control based on field samples or models (e.g., Firestone et al., 2007, 2010a; Bunch et al., 2012; Israde-Alcántara et al., 2012, Kennett et al., 2009a, b; LeCompte et al., 2012; Wittke et al., 2013a; Wu et al., 2013). From among these publications, the dating of 29 sites was evaluated by Meltzer et al. (2014).
Again, the most comprehensive and recent investigation of the dating of YDB sites is by Kennett et al. (2015a). As already stated, Meltzer et al. (2014) is fundamentally flawed, as already highlighted by Sweatman (2021).
HEA: As summarized in their abstract (p E2162) “Several of the sites lack any age control, others have radiometric ages that are chronologically irrelevant, nearly a dozen have ages inferred by statistically and chronologically flawed age–depth interpolations, and in several the ages directly on the supposed impact layer are older or younger than ~12,800 calendar years ago. Only 3 of the 29 sites fall within the temporal window of the YD onset as defined by YDIH proponents.” Further, Meltzer et al. (2014, p E2169) note “We even relaxed one of their criteria, namely that ‘only 14C dates with measurement precisions <100 years, and preferably <60 years, should be used’ in assessing the supposed impact chronology and its potential effects [Kennett et al., 2008b]. Had we applied it, we would have had to discard all luminescence ages and almost 60% of all radiocarbon ages used by YDIH proponents. Doing so would have instantly removed all radiometric age control from 11 sites and left 8 more with only a single age that in no case dates to the YD onset, meaning that 19 of their 26 sites with radiometric ages (group 1b) would become essentially free floating chronologically.” ENDNOTE 6
HEA: The approach taken by Meltzer et al. (2014) was criticized by Sweatman (2021, p 15-16, 20). He wrote “no standard errors were provided for their calculations. It is therefore not possible to determine if any of these age differences are significant. In a technical sense, therefore, their data is meaningless and their conclusions cannot be supported” (emphasis added). This comment misses several key points and is factually untrue.
Sweatman (2021) was referring to the absence of any uncertainty reported in the coefficients of Meltzer et al.’s (2014) linearly regressed age-depth models, and thus also in their calculations for the age of each YDB site. His statement is therefore factually true. The lack of such error estimates in Meltzer et al. (2014), along with HEA’s support for their omission, is at odds with best practice in science.
HEA: The text (Meltzer et al., 2014, p E2167-E2168) includes discussion of error and uncertainty and the Supplemental Data clearly includes the standard errors in their calculations for all 29 YDIH sites reviewed.
This is highly misleading. Standard errors are provided for individual radiocarbon measurements but not for the coefficients of any lines of regression fitted to this data. Thus Meltzer et al.’s (2014) conclusions are unsupported.
To be clear, the full list of tables and figures in the supplemental information of Meltzer et al. (2014) is as follows. Tables and Figures 1–16 list individual measurements for YDB sites. Table 17 lists model data for their alternative age-depth modeling. Table 18 lists the regression coefficients for their regression lines for each site, but no uncertainty estimates in these regression coefficients are provided. Table 19 lists r2 and p values for their regression lines, but this has little to do with uncertainty estimates for the regression coefficients.
In fact, the r2 value is an indication of how well a linear fit represents the underlying data. In other words, it’s a measure of the scatter in the data relative to the fitted line. Although this is related to the uncertainty in the linear coefficients of the fitted line, it is not the uncertainty measurement itself. In any case, Meltzer et al. (2014) ignore these r2 values in making their conclusions. Moreover, the p-value is a measure of the probability that the linear model actually reflects a trend in the data, i.e. is there actually a linear trend or is the trend line spurious? Once again, this is only tangentially related to the uncertainty in the regression line coefficients. Ultimately, Meltzer et al. (2014) make no use of these statistical measures in their conclusions, which in any case are not the uncertainty measures needed.
Indeed, simple inspection of the scatter in the underlying data in many of Meltzer et al.’s (2014) plots shows that there is considerable uncertainty in these regression lines that likely far outweighs the uncertainty in the date of the YDB provided by Kennett et al. (2015a). It is therefore crucial that it is considered.
HEA: The ages just had to be fully outside the range of the YD/GS-1 onset age (~12.9 cal ka BP) to show that the markers of a YDB impact did not occur in the profile when/where they were supposed to occur.
This is false. In fact, Meltzer’s (2014) age-depth modeling and analysis is flawed in two fundamental ways. First, they take no account of the uncertainty in their fitted regression lines, as already mentioned. Second, they make age-depth models and perform regression modeling using calibrated age estimates rather than raw C14 radiocarbon measurements. The importance of this latter issue was already discussed in our Section 4 in relation to the age-depth model of Gill et al. (2009).
This point is illustrated in Figure 4 below using Meltzer et al.’s (2014) age-depth data for Abu Hureyra (see their supplemental data). We use the raw C14 radiocarbon measurements provided in Table S1 of Meltzer et al. (2014) (blue symbols plotted with 1-sigma error bars). Weighted (red line) and unweighted (blue line) linear regression models using the method of least squares are also shown. Meltzer et al. (2014) instead provide linear fits (weighted and unweighted) to the calibrated radiocarbon data. The weighted regression line takes account of the intrinsic radiocarbon error measurements. We find that most of the radiocarbon measurements fall outside of 1 standard deviation (sigma) from the red regression line using the intrinsic measurement uncertainty. This strongly indicates that the radiocarbon measurement error bars do not fully account for all the uncertainty in this data (as mentioned in our Section 4, this is not unexpected). Therefore, it is more appropriate to use an unweighted fit and to use the standard deviation of the radiocarbon measurements relative to this unweighted regression line to estimate the true measurement error. This is found to be 300 radiocarbon years for each data point. Using this new measurement uncertainty, the orange lines added in Figure 4 depict linear fits that are within 2-sigma (95% probability using a normal distribution) of the unweighted best fit line. That is, they define a 2-sigma (95%) envelope of uncertainty in the best fit line. Meltzer et al. (2014) neglected to include these lines or any other analysis of the uncertainty in their linear fits. Even if they had, their method would have been erroneous because they used the calibrated radiocarbon data instead.
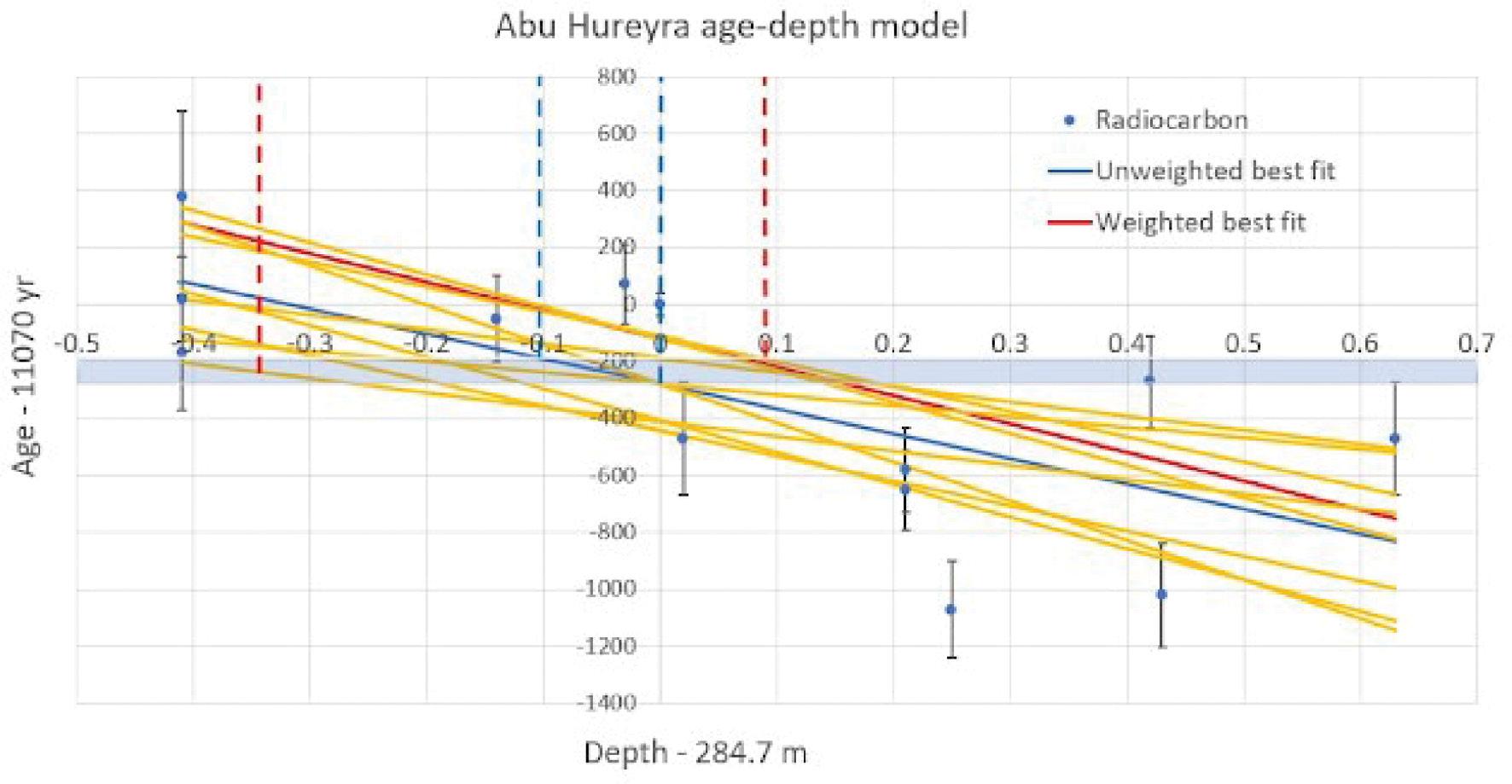
Red and blue lines are linear least-squares fits to C14 radiocarbon data for Abu Hureyra. The orange lines indicate the uncertainty envelope (at 2-sigma) in the age-depth relationship based on the unweighted best fit. Lines such as these were neglected by Meltzer et al. (2014) but are crucial in modelling the uncertainty in this data. See the text for a description of the vertical dashed lines.
Meltzer et al. (2014), supported by HEA, effectively argue that YDIH proponents need to show that the position of the YDB in the sediment is between the blue dashed vertical lines above, since these represent the (2-sigma) uncertainty in the date of the YDB (represented by the horizontal blue bar) against the (blue) unweighted regression line. But this neglects the uncertainty in the regression line itself, illustrated by the envelope of the orange lines. When this uncertainty is taken into account, YDIH proponents need only to show the position of the YDB in the sediment is between the red dashed vertical lines, a much less demanding requirement (at 2-sigma). The depth of the YDB at Abu Hureyra is stated to correspond to 284.7 meters above sea level (masl) (Wittke et al. (2013) and Moore et al. (2020) but not Bunch et al. (2012)). We see from the above plot that this depth is only marginally consistent at 2-sigma with the age of the YDB determined by Kennett et al. (2015a) when the regression uncertainty is omitted (i.e. the blue lines only just straddle 284.7 masl). However, it is easily consistent at 2-sigma with the age of the YDB determined by Kennett et al. (2015a) when the regression uncertainty is included (i.e. the red lines easily straddle 284.7 masl). Thus, the regression uncertainty must be considered because it is much larger than the YDB age uncertainty. But Meltzer et al. (2014) fail to take this uncertainty into account for every site they analyze (and, in any case, they used the calibrated radiocarbon data instead, which is erroneous). Thus, their conclusions are unsupported.
The above analysis is appropriate for the simple kind of regression modeling (linear fitting) employed by Meltzer et al. (2014). Kennett et al. (2015a) used a more sophisticated Bayesian modeling technique for dating YDB sites, i.e. their models include additional information such as assumptions about the time-order of the samples. In principle this can be a better approach, but appropriate care must be taken in interpreting the results.
HEA: The issue is not whether their results and the cases they re-analyzed were significantly different. Further, in the main text and the Supporting Data, Meltzer et al. (2014) provide ample discussion of their methods and their statistical significance.
The only values relating to statistical significance provided by Meltzer et al. (2014) are the r2 and p values provided. These have little to do with the uncertainty in the regression parameters as stated above. In any case, these statistical measures are not used by Meltzer et al. (2014) in framing their conclusions.
HEA: Like the alleged issue of incorrect sampling at Arlington Canyon (Section 4.1), Sweatman (2021) and other YDIH proponents never address the problematic nature of many of the samples, sample contexts, and resulting dates. In a technical sense, therefore, Sweatman (2021) simply dismisses a carefully laid out analysis using an irrelevant technicality.
HEA describe Meltzer et al.’s (2014) analysis as ‘careful’ and the required regression coefficients as an ‘irrelevant technicality’. In fact, the opposite is true, as shown above. Kennet et al. (2015a) made similar criticisms of Meltzer et al. (2014), but they have been ignored by YDIH opponents, including HEA.
To show that any of the “samples, sample contexts and resulting dates” are problematic, HEA should perform competent age-depth modeling for each site in question that takes into account the uncertainty in their fitted regression lines. Otherwise, such claims can be regarded as spurious.
HEA: Sweatman (2021) similarly criticizes a box plot (from Holliday and Meltzer 2010, figure 3; Holliday et al., 2014, figure 2) of radiocarbon dates on the “black mat” (Section 6). Sweatman (2021, p 16) comments that “much of the data in this plot is considered unreliable or is unpublished.” ENDNOTE 7.
HEA: All data in the figure are directly from the published citations in Holliday and Meltzer (2010, figure 3 caption). The integrity of those dates can be evaluated from the information within those cited sources. However, Sweatman (2021) does not elaborate on his perceived unreliability of “much of the data.” Only two examples of problematic dates are offered, Naco and Wilcox. The Naco date was included inadvertently in Holliday et al. (2014) and should be discarded.
Most of this data is originally from Haynes (2008) who considered many of the dates unreliable. For example, Haynes (2008) states;
“From SI Table 2 (and see Fig. 5) it is apparent that the quality of YD black mat radiocarbon dating needs improvement especially near the upper and lower boundaries.”
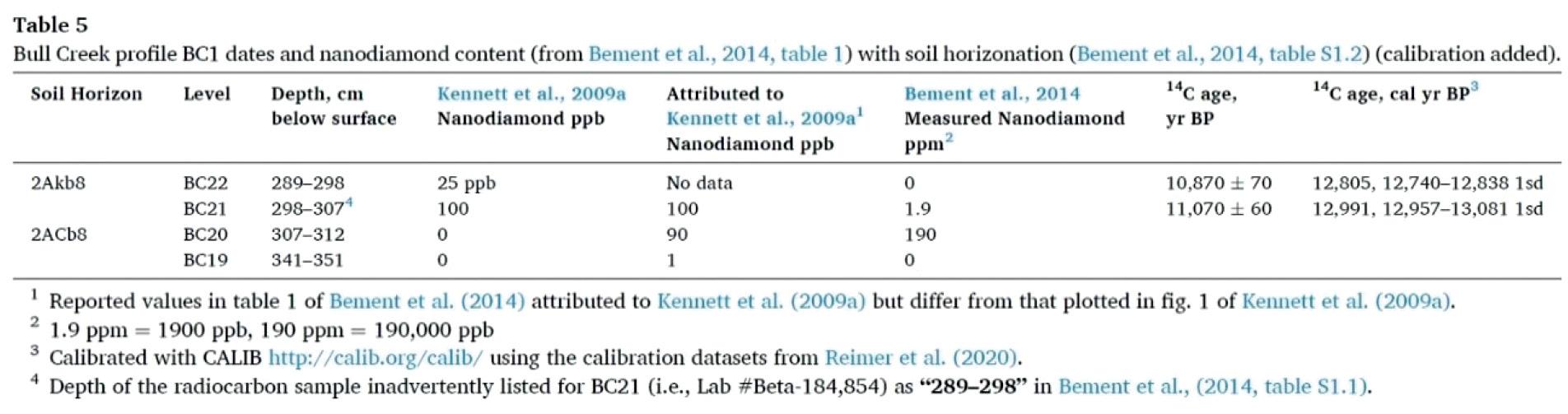
Table 5 from HEA. For unknown reasons, HEA inappropriately shifted the 4th column (Kennett et al…) upwards by one cell.
In SI Table 2 of Haynes (2008) over 40% of black mat sites are characterized by at most one radiocarbon measurement, and it is unclear how many of the measurements correspond to the base of the YD black mat where we expect to find impact proxies (if they exist at all at that site). Thus, the data in these figures is of little practical relevance to this debate.
HEA: Sweatman’s discussion of the dating of the Willcox section provides further circular reasoning (Table 2).
The issue here is the age of the YDB at the sites in Figure 3 of Holliday and Meltzer (2010). Within the YDIH model, the YDB is consistently defined by the location of impact proxies. Often, but not always, these indicators are found at or near the base of the YD black mat. Indeed, Haynes (2008) writes;
“This layer or mat covers the Clovis-age landscape or surface on which the last remnants of the terminal Pleistocene megafauna are recorded. Stratigraphically and chronologically the extinction appears to have been catastrophic, seemingly too sudden and extensive for either human predation or climate change to have been the primary cause. This sudden Rancholabrean termination at 10,900 +- 50 B.P. appears to have coincided with the sudden climatic switch from Allerød warming to Younger Dryas cooling … Therefore, the Z1–2 contact [i.e. the YDB] appears to be the same age everywhere, essentially isochronous, in this study (Fig. 5).”
As for Table 1, except showing only putative ‘super-Tunguska’ impact sites.
Country | Site | Date BP | 2σ | Refs |
---|---|---|---|---|
Canada | Lake Hind | 12745 | 360 | Kennett |
Chile | Pilauco | 12770 | 320 | Pino et al. |
Venezuela | Mucunuque | 12787 | 60 | Mahaney |
EU, Netherlands | Aalsterhut | 12780 | 70 | Kennett |
Syria | Abu Hureyra | 12825 | 110 | Kennett |
US, CA | Arlington Cyn | 12805 | 110 | Kennett |
US, OK | Bull Creek | 12840 | 150 | Kennett |
HEA provide no evidence that YD impact proxies are located at other depths within the YD black mat. The caption to Figure 3 of Holliday and Meltzer (2010) reads; “Box plot showing calibrated ages (square) and 1 standard deviation (vertical bars) for lowest or oldest (or only) “black mats””. As we are specifically interested in the age of impact proxies near the base of the YD black mat, it is not clear that any of this data is relevant to the debate.
As an example, for the Wilcox Playa site the radiocarbon sample is taken from near the top of the YD black mat and is described as the “minimum age” of the YD black mat by Haynes (2008, SI Table 2). Thus, Holliday and Meltzer’s (2010) date for the Wilcox Playa site in their Figure 3 is irrelevant.
Once again, HEA do not explain why Sweatman’s reasoning is circular, and for the same reasons that we rejected the accusation of circular reasoning applied to the interpretation of Gill et al.’s (2009) data in our Section 4, we reject it here too. If every opportunity to test the YDIH is rejected by HEA on the grounds of circular reasoning, then HEA’s viewpoint will clearly be a self-fulfilling prophecy. This single point illustrates a key problem with HEA’s approach; they lack the logic to properly assess the YDIH. In fact, any hypothesis must be tested against its own claims. HEA consistently fail to do this.
HEA: Besides, Kinzie et al. (2014, p 478) cite unpublished data from three sites with nanodiamond horizons purported to support the YDIH and employ circular reasoning (Table 2).
It is not clear to which entry in Table 2 of HEA this remark refers. But two sites from Kinzie et al. (2014) are mentioned in Table 2; Gainey and Chobot. Both are discussed in detail in Wittke et al. (2013, Appendix) and both boundary layers contain geochemical impact markers. At Chobot the position of the YDB is inferred from terminal Clovis artifacts, which is consistent with the YDIH. At Gainey, the site is dated by a single OSL measurement at 12.36 +- 1.23 ka, which is also consistent with the expected date of the YDB. Moreover, artifacts from what is interpreted to be a marginally post-Clovis culture appear in the sediment slightly above the inferred YDB. Both sites are recognized to be of low dating quality by Kennett et al. (2015a), but both sites are nevertheless consistent with the YDIH.
Once again, there is no circular reasoning here, and once again, HEA do not assess the YDIH on its own terms. They confuse circular reasoning with a consistent test of the hypothesis and the application of Occam’s razor, or parsimony, which is the central dogma of the scientific method. With logic like HEA’s it is impossible to test the YDIH properly, and thus their viewpoint is a self-fulfilling prophecy.
HEA: Shortly after the publication of the dating critique by Meltzer et al. (2014), Kennett et al (2015a) published a paper on a Bayesian chronological approach for estimating the ages of claimed YDB zones from many of the sites examined by Meltzer et al. (2014). Thirty-two sites are listed and discussed. The dating at nine is of such poor quality that Kennett et al. (2015a) could not include their results, but they were still claimed to be YDB via circular reasoning (Table 2).
For the same reasons as before, there is no circular reasoning here. These sites (including Gainey and Chobot discussed above) are consistent with the predictions of the YDIH and they support the YDIH via the principle of parsimony. As already stated, their frequent claim of “circular reasoning” is an anti-scientific rhetorical device that attempts to isolate individual data points that support the YDIH. Their approach is a corruption of the scientific method.
HEA: In a brief response to a critique of their Bayesian modeling, Kennett et al. (2015b, E6723) dismiss the criticisms by stating that these “same claims previously were presented in Meltzer et al., [2014] and were discussed and refuted in Kennett et al., [2015a] …” But in fact, few of the criticisms enumerated by Meltzer et al. were even addressed by Kennett et al. (Table 1).
As shown above, Meltzer et al.’s (2014) work is fundamentally flawed and therefore its claims are unsupported.
HEA: YDIH proponents (e.g., Wolbach et al., 2018a, b; Sweatman 2021; Mahaney et al., 2022; Powell 2022) largely accept the work of Kennett et al. (2015a), suggesting that the YDB is synchronous across four continents, and thereby assert that the impact indicators were deposited synchronously over four continents.
This is misleading. The claim in Kennett et al. (2015a) is that the dating of the YDB on four continents is not inconsistent with the hypothesis. Synchroneity is the most likely explanation for this, as discussed by Sweatman (2021), but we will come to this argument in detail later.
HEA: Mahaney et al. (2022, p 17) states that the conclusions of Meltzer et al. (2014) were “refuted using Bayesian statistics by Kennett et al., [2015a].” But like Kennett et al. (2015a), those proponents fail to recognize or refute the identification of many problems with the original site contexts of the dating discussed in detail by Meltzer et al. (2014; with over 60 pages of text and tables) and by Holliday et al. (2014, 2020).
To show that any of the “problems with the original site contexts of the dating discussed in detail by Meltzer et al.” are significant, opponents of the YDIH should construct competent age-depth models that show they have a significant impact on the calculated age of any YDB site. Because their work is fundamentally flawed, as shown above, Meltzer et al. (2014) were unable to do this.
HEA: Sweatman (2021, p16) focuses on eight “high-quality sites” (using the classification of Kennett et al., 2015a for ranking the chronologies at the 23 sites included in the age estimate). But several of these high quality sites are problematic. Six were claimed to produce “radiocarbon dates from directly within the YDB layer” (Kennett et al., 2015a, table 1). However, one date is from the Bull Creek site, where the radiocarbon date is stratigraphically above a claimed “impact indicator” spike (Section 5.5, Table 5) and, ironically, YDIH proponents often use such spikes to identify the YDB (Table 2).
HEA: Two dates (from Barber Creek and Blackville) have standard deviations >700 years (i.e., very poor precision) and thus no evidence whatsoever that the claimed YDB zone is of YDB age.
HEA: Two other dates (from Aalsterhut, Lingen) are for the Usselo soil in northern Europe and of YDB age but selected from among scores of dates for dozens of sites falling far outside of the YDB (e.g., Hoek 1997; Kaiser et al., 2009) (further discussed in Section 5.6). Picking out dates that are conveniently YDB age has no relevance to the YDIH debate and, moreover, is scientifically unsound.
HEA: These examples of just the so-called “high-quality sites” well demonstrate that statistical analyses, Bayesian or otherwise, cannot overcome poor sample context, selection or precision, previously published flawed age–depth interpolations, or unexplained and inappropriate rejection of published dates. This is a classic example of the use of poor data resulting in the production of poor statistical results. ENDNOTE 8
HEA have not shown that the dating of any YDB site is inconsistent with the YDIH. An argument for synchroneity of YDB sites is provided later in this section.
The table in Figure 5 above presented in HEA refers to nanodiamond (nd) measurements and radiocarbon samples collected from the Bull Creek site by Kennett et al. (2009) and Bement et al. (2014). However, the data for Kennett et al. (2009) has been inappropriately shifted upwards by one cell by HEA. In fact, the measurement of 25 ppb should be in BC21 and the measurement for 100 ppb should be BC 20. This is confirmed in the text of Bement et al. (2014);
“Kennett and colleagues (4) found a concentration of nds (both n- and cubic forms) centered on the boundary between two soil A horizons interpreted to be the YDB and equivalent to our samples BC20 and BC21.”
It is not clear why HEA shifted these results upwards by one cell, but we expect this is the reason for their confusion. Clearly, when critiquing work it is imperative that the original data is not misrepresented. Any study can be “refuted” if its data is mistreated. This data is discussed again below in HEA’s Section 5.5.
HEA: Sweatman (2021, p 16) goes on to discuss standard deviations of the modeling results of Kennett et al. (2015a). He seems satisfied with modeled results at 2 or 3 standard deviations (sd) confirming a YDB age for a sample zone. But such statistical confirmation has nothing to do with stratigraphic or chronologic reality. An age model uncertainty of 100 years (1 sd) means that the age of a sample at 2 sd would be within a range of 400 years (or 800 years at 3 sd). Such broad age ranges cannot confirm the identification of a moment in time in the stratigraphic record.
Actually, an uncertainty of 100 years at 3 sd would be within a range of 600 years, not 800 years. In any case, all that YDIH proponents need to show is that the age of any given YDB site is not inconsistent with the age of the YD impact at some reasonable level of confidence. Demanding that the age range should “confirm the identification of a moment in time in the stratigraphic record” is a meaningless unscientific statement that fails to understand very basic concepts in data analysis.
HEA: The ages of the authors of this commentary could be modelled to statistically date to the signing of the Declaration of Independence (1776 CE). The modelling could be statistically correct, but obviously meaningless.
HEA: Further problems with the results of the Bayesian age estimation are enumerated by Holliday et al. (2020, p 70-71, 75). “Modeled age ranges with standard deviations of >300 years up to 2405 years are presented for layers of claimed impact indicators at nine sites of ‘low quality’ in terms of dating (their description in Kennett et al., 2015a, table 2). These layers are argued to represent the YDB based solely on the premise that if they could be YDB, they must be the YDB” (Holliday et al., 2020, 70-71, table 5) (Table 2).
No YDIH proponent has made this argument. It is an invention of HEA. However, the proposal that the YDB is very likely synchronous on four continents can be made using a statistical argument, presented later.
HEA: Their conclusion also suggests that the modeled dating of 12,255 +/− 2405 cal yr BP for the sample zone at Melrose is somehow proof of a YDB age, which is of course preposterous.
As already stated, the proposal that the YDB represents a synchronous event can be made using the statistical argument provided below. Melrose has impact proxies similar to other YDB sites that are not inconsistent with the age of the YD impact event. Therefore, Melrose is a potential YDB site until shown otherwise. HEA do not show this.
HEA: There is also a continuing and troublesome problem of omitting radiocarbon ages without explanation or analyses, which is a problem in much of the YDIH literature (enumerated by Meltzer et al., 2014; Boslough et al., 2015; Holliday et al., 2020) (Table 4).
None of the cited literature demonstrates that any of these omissions are problematic. As stated earlier, to show they are problematic, competent age-depth models that omit any supposed problematic data points should be produced and compared. Meltzer et al. (2014) attempted this, but as shown above their understanding of how to produce these regression models, especially their inherent uncertainty, is flawed. Thus, HEA provide no evidence any of these supposed problems are actually significant.
HEA: Sweatman (2021) offers several contradictory and inconsistent concluding statements of sorts regarding the dating of the YDB. “No YDB site has yet been found to be obviously inconsistent with a synchronous event circa 10,785 ± 50 cal BP (2 sd).” (p. 19; see also p 17).
Apart from the typo (the date should be 12,785), Sweatman (2021) is correct when one takes into account a proper treatment of the uncertainty in the YDB site regression modeling. See the example for Abu Hureyra above.
HEA: Besides the problematic date notation and apparent typographical errors (Section 5.1), this statement is a non sequitur. The YDB of the YDIH, especially when it is claimed to contain “impact markers,” must (by definition) represent a synchronous moment in time, dated to ~10,785 14C yr BP.
This statement is scientifically meaningless as no uncertainty range is provided by HEA. Moreover, HEA do not state how they obtain this uncalibrated date or provide a citation.
HEA: To be a “YDB site” the site must clearly contain a zone accurately dated to the YDB with high precision.
This is false. To be a potential YDB site it should i) contain a zone with impact markers consistent with those already found at other YDB sites, and ii) have a date that is not obviously inconsistent with the YD impact or be found with other proxies that are predicted to indicate the YDB, such as terminal Clovis artifacts.
HEA: As pointed out above and in Sections 5.4 to 5.7, and Table 4, many claimed YDB zones and evidence for a synchronous “event” are not so or are not clearly shown to be so.
An argument for synchroneity of the YDB is given later. YDIH opponents have not shown that any of the YDB sites are obviously inconsistent with the suggested YD impact age.
HEA: He also comments that dating at eight “high-quality sites” among the 23 dated “is consistent with a synchronous event, which suggests all YDB sites are likely synchronous” and “it would be surprising if the others were not all eventually found to also be consistent” (p 16). These are baseless assertions that defy fundamental principles of objective science.
Sweatman’s implicit assertions were based on an obvious statistical argument which is provided explicitly later. It shows that the YDB very likely represents a singular event or a series of highly correlated events.
HEA: Taken to its logical conclusion, this assertion argues that there is no longer a need to date archaeological sites or geologic sections. We can just assume high-precision dating by correlation.
HEA: Given that the burden of proof is on the YDIH proponents, dating results from one site or a group of sites does not confirm the dating at others. It only provides testable hypotheses, of the kind evaluated by Jorgeson et al. (2020) (Section 5.8).
The argument about the synchroneity of the YDB across four continents is actually very simple. Impact proxies are found at over 50 YDB sites on four continents. In each case the evidence indicates significant disturbance (melting, vaporization etc.) of the ground, although no craters of YDB age are yet confirmed. Therefore, this evidence suggests a multitude of low-altitude airbursts distributed across several continents with a wide range of impact energies. Some were likely much larger than Tunguska, i.e. super-Tunguskas, because of the presence of significant melt glass at these sites. Note that large chunks of melt glass are not apparent at Tunguska.
It’s important to realize that the usual background rain of cosmic dust or the burn up of small meteorites at high altitude or even high-altitude airbursts cannot explain the YDB debris, since it mainly has a similar composition as Earth’s crust, although some meteoric material is sometimes present. Therefore, only major, low-altitude airburst or ground impacts can explain the YDB debris.
Summarizing the data for all YDB sites so far, we find the following;
8 sites consistent with the YD impact date with uncertainty (95%) < 200 years
10 more sites consistent with the YD impact date with uncertainty (95%) < 400 years
9 more sites consistent with the YD impact date with uncertainty (95%) < 1600 years
These sites are shown in Table 1 below.
HEA suggest that the Tunguska event was a 1 in 1000 to 1 in 10,000-year event. Clearly, the probability of so many Tunguska-like impacts within such a relatively short time span suggests it is extremely likely that that the YDB impact proxies were generated synchronously by a single impact event or a series of related and highly correlated events within a short time span, i.e. the probability of these being independent Tunguska-like events (the null hypothesis) is extremely low.
This reasoning can be made statistically robust using a Poisson distribution. Let’s suppose that 1.0 Tunguska events are normally expected within 1000 years, conservatively taking the higher impact rate stated by HEA for Tunguska-like events. Inspection of Table 1 shows that 17 YDB sites fall within the range 12,280 – 13,270 cal BP, i.e. approximately a timespan of 1000 years using the 2-sigma confidence interval for each site. Using the Poisson distribution, the probability of finding at least 17 such events within a 1000-year interval is 1.1 × 10−15, which is negligible.
Even if we allow for a much higher impact rate of Tunguska-like impactors, say 2 every 1000 years, we still find a vanishingly low probability of 5.0 × 10−11. Thus, a multitude of unrelated Tunguska-like impactors cannot reasonably explain the YDB.
Now let’s consider another impact model. Let’s instead suppose all the YDB impact debris was created by several independent super-Tunguska airbursts that interact strongly with the ground, each with a supposed impact rate of 1 in 10,000 years, that can distribute impact debris (microspherules, nanodiamonds, platinum) over several thousand km. We would need a super-Tunguska near Abu Hureyra to account for the melt-glass found there. Three more are needed in Western Europe, Central America and South America to account the impact debris there. Another is needed on the East Coast of the USA to account for the melt-glass there, another on the West Coast of the USA to account for impact debris there and another near Chicago to account for the northern US debris. The date resolution for each of these sites corresponds to the lowest age range of all the associated YDB sites covered by each super-Tunguska impact. Table 2 below shows the resulting data.
Again, using the Poisson distribution the probability of finding at least 7 such events within a period of 720 years is 1.9 × 10−12. Therefore, we can discount this scenario too. Even if we use double the impact rate for these large, low-altitude airbursts we still have a probability of 2.2 × 10−10.
Furthermore, YDB sites appear to be relatively common. Therefore, we can expect the final number of YDB sites to number in the hundreds or thousands, not just ~ 50. Thus, the probability estimates above will almost certainly be too large by very many orders of magnitude.
Essentially, there is no realistic possibility that a common layer of impact proxies dated to within a thousand years across four continents can signal anything other than a singular impact event, or highly correlated series of events.
As a check, let’s consider a YDIH impact model which posits a single event, or series of highly correlated events in a short time span, with a total magnitude of at least 10,000 Mt, which corresponds to ~ 1000 Tunguskas or 10-100 super-Tunguskas, which is an impact scale consistent with current evidence for the YDIH. According to Figure 2 in Boslough et al. (2012), shown below in Figure 6, such an event is expected to occur about once every 200,000 years. Therefore, the probability of one such event in the last 13,000 years is about 0.06. This is quite reasonable (within 2-sigma) and should be vastly preferred to the other models discussed above. However, the figure below shows the expected average impact rate over the last few hundreds of million to billion years. Impact rate fluctuations, i.e. coherent catastrophism, can be expected on shorter timescales when giant comets intrude into the inner solar system. Indeed, a significant impact rate increase is expected in recent millennia given the existence of the very large and old Taurid meteor stream and the relatively dense zodiacal dust cloud. This would make the Younger Dryas impact a reasonably probable event. This view is consistent with Napier (2010) and Moore et al. (2024).
6.2. HEA’s Section 5.4. Poorly Dated Platinum Anomalies
HEA: Platinum (Pt) anomalies are used by YDIH proponents to unquestioningly support the YDIH (see Section 11). The Pt anomaly in the GISP2 ice core (Petaev et al., 2013), which is proclaimed by Sweatman (2021, p 20) to be “probably the most significant [YDIH] evidence so far” post-dates the onset of the YD/GS-1 (see Section 5.1).
Here, HEA define the onset of the YD period using Steffensen et al.’s (2008) criterion, which corresponds to a large fluctuation in the deuterium excess (d) in the NGRIP ice core. However, this definition is contentious for several reasons. First, this fluctuation in d is not known to occur in any other ice core and therefore could simply be an artifact of the NGRIP ice core. Second, it is not clear that large fluctuations in d necessarily indicate major and sudden changes in climate at that time. For example, Lewis et al. (2013) state,
“… although deuterium excess is generally a faithful tracer of source temperatures as estimated by the MCIM approach, large discrepancies in the isotope-climate relationship occur around Greenland during the Last Glacial Maximum simulation, when precipitation seasonality and moisture source regions were notably different from the present. This identified sensitivity in d as a source temperature proxy suggests that quantitative climate reconstructions from deuterium excess should be treated with caution for some sites when boundary conditions are significantly different from the present day.”
Wolbach et al. (2018a) also objected to the use of Steffensen et al.’s (2008) criterion,
“Steffensen et al. (2008) measured five different proxies for climate change and obtained five seemingly contradictory dates for the YD onset, from oldest to youngest: (1) 12,896 ± 1.5 cal BP for deuterium excess (d), a proxy of past ocean surface temperatures; (2) 12,787 ± 24 cal BP for annual layer thickness (l), a measure of the rate of snowfall; (3) 12,737 ± 8.9 cal BP for calcium ions (Ca21), a proxy for source strength and transport conditions from terrestrial sources; (4) 12,735 ± 8.9 cal BP for dust concentrations, a proxy for source strength and transport conditions from terrestrial sources; and (5) 12,712 ± 74 for d18O, a proxy for past air temperature at the site. Regarding these dating discrepancies, Steffensen et al. (2008) consider that the oldest date, 12,896 ± 1.5 cal BP, is the actual date of YD onset and that the younger dates are due to lags in the response times of the other proxies. All of the above age differences affect the reliability of conclusions that use ice-core data.”
HEA: Moore et al. (2017) report a widespread Pt anomaly at the YDB in 11 sites across North America. Powell (2022, p 24) and Sweatman (2021, p 17) uncritically accept those interpretations, the latter going so far as to conclude that the Pt zone can be used to “unambiguously identify the Younger Dryas boundary at many locations around the globe.” Unfortunately, the dating reported by Moore et al. (2017) suffers from many of the same problems of chronology that plague the original YDB sites in Firestone et al. (2007) (Section 5.3) (Table 4). As is obvious from Moore et al. (2017, Supplementary Information), only three sites seem to have direct dating for the Pt anomaly, but only near the YDB (Arlington Canyon; Murray Springs, Blackwater Draw). However, Arlington Canyon shows considerable vertical and lateral facies variation (Section 4.1). As for the other sites, five are indirectly inferred dates based on archaeology (two of which are based on Optically stimulated luminescence (OSL) with large standard deviations), two have no numerical age control whatsoever, and one (Sheriden Cave) has a very confusing geochronological context (Table 4).
Petaev et al. (2013) discovered a platinum anomaly in the GISP2 ice core that is synchronous with a clear change in Greenland’s climate at that site. They suggested it was likely generated by a cosmic impact and given its breadth within the ice core they predicted a global platinum anomaly. Later, Moore et al. (2017) found a platinum anomaly exactly where it is predicted to occur at 11 YDB sites. The prediction is that it should occur in the same debris layer as an abundance of other impact proxies and be consistent with the date of the YD impact event or be correlated with terminal Clovis artifacts, and this is confirmed in every case.
In our Section 6.1 above we argued that impact markers at 17 sites on 4 continents were almost certainly synchronous considering that all these sites are dated to within a 1000-year window. So far, excess platinum has been detected whenever it has been sought at 8 of these well-dated sites in combination with at least one other impact marker. Thus, the YDB platinum anomaly can be used as a more precise method for dating artifacts than radiocarbon dating as it should mark the exact position of the YDB. The same principle is used by the discipline of tephrochronology where volcanic tephra layers are used to correlate and synchronize subsurface stratigraphy over long distances (Lowe 2011).
HEA: Repeating Moore et al.’s (2017) claim, Powell (2022, p 24) states that the Pt anomaly at three sites (Murray Springs, Blackwater Draw, Sheriden Cave) is “associated with Clovis artifacts, representing the level at which the Clovis culture disappeared” and other sites in the southeastern U.S. “are poorly or not directly dated and lack the black mat, but do provide a coherent Clovis archaeological record.” Sweatman (2021 p 5) highlights the Pt anomaly at “the Flamingo Bay site in South Carolina” claiming “a platinum abundance nearly 100 times the average crustal value was found in association with the youngest Clovis artefacts.” But the context of the archaeology and the Pt zones is both mixed and confused, Table 4 and Moore et al. (2017, p 6). Setting aside the YDIH requirement that the YDB represents the termination of Clovis, a basic tenant of many versions of the YDIH (Section 3.1), …
This is misleading. The instantaneous termination of Clovis is not a prediction of the YDIH. Instead, the YDIH predicts the YD impact was the beginning of the end for Clovis, and various lines of evidence support this. See also HEA’s Section 5.7 below.
HEA: … and the likelihood that the “youngest Clovis” would be post-YDB (Waters and Stafford 2007, Waters et al., 2020), …
To show that the “youngest Clovis” is post-YDB it is necessary to show that Clovis artifacts occur above the YDB. in fact, no Clovis artifacts have ever been found above the YDB. Section 5.7 of HEA below provides more details of this debate.
HEA: … both authors neglect to note this comment from the main text in Moore et al. (2017, p 6) “Early Archaic artifacts in the same levels as Clovis at Flamingo Bay” indicate that “these surfaces were stable to slowly accreting for several millennia before being buried incrementally through a combination of slopewash and aeolian accretion…” The authors seem to believe the self-contradicting notion that a mixed archaeological assemblage spanning thousands of years of Clovis and Early Archaic time somehow provides a precise stratigraphic age indicator for the YDB.
HEA are mistaken; there is no contradiction here. The YDIH predicts that at each site the YDB (which is located by impact proxies, including platinum) should be consistent with the suggested age of the YD impact or correlate strongly with the youngest Clovis artefacts. Moore et al.’s (2017) data from Flamingo Bay is not inconsistent with this prediction. A mixed assemblage can result from a number of taphonomic processes, but the presence of the platinum in combination with Clovis artifacts indicates some degree of preservation of the YDB. To refute this aspect of the YDIH, opponents need to show that Clovis artifacts are found consistently above the YDB, and this has never been found to occur.
HEA: Further, Moore et al. (2017, Supplementary Information, p 5) observe “Many sandy sites in the eastern US contain Paleoindian and Early Archaic components within the same stratigraphic zone or with very little separation (e.g., Topper, Kolb, and Flamingo Bay). As a result, Pt anomalies may be expected to occur in some sites within stratigraphic sequences that contain both Paleoindian and Early Archaic artifacts or with Early Archaic artifacts sitting immediately above YD-age sediments. Archaeological occupations at Squires Ridge, beginning with Early Archaic side-notched stone tool industries, are found only within and above the deepest Pt anomaly and only pre-cultural, archaeologically sterile zones lie underneath the deepest Pt anomaly. This is consistent with post-depositional processes and reworking of Pt enriched sediments during periodic landform aggradation events during and after the YD event” (emphasis added). This astonishing admission demonstrates that the Pt zone is mixed among Early Archaic (post-YD/GS-1) artifacts and thus does not represent a discrete stratigraphic context, nor (contrary to Powell, 2022, p 24) a “coherent Clovis archaeological record” (Table 4).
For the same reasons as above, HEA are mistaken. There is no contradiction with the YDIH here. Far from astonishing, the reworking of stratigraphic layers due to taphonomic processes is commonplace and does not invalidate the use of the Pt as a datum.
HEA: This conclusion is yet another among publications where dating is based on assuming that because a zone could be YDB in age, it must be the YDB (Table 2).
This is false, as no YDIH proponent has claimed this. It is an invention of HEA. The requirement for classification of any site as a YDB site was stated earlier several times. That is, any site with impact proxies, including platinum, can be considered a YDB site if its age is not inconsistent with the suggested age of the YD impact or if it correlates strongly with terminal Clovis artefacts. YDIH proponents are consistent in applying these criteria.
Moreover, the statistical argument given earlier shows the only reasonable interpretation is that the YDB signals a singular event or highly correlated series of events.
HEA: In other words, there is no clear age control. Given the dating problems noted above, the reference to “widespread Platinum abundance in bulk sediments near the base of YD-age black mats on at least four Continents, confirmed by several independent research groups” (Sweatman 2021, p 17) is not supported by the evidence.
As we have shown above, HEA’s view is not correct. Platinum is always found in the YDB with other impact proxies and in every case its age is not inconsistent with the suggested age of the YD impact or it correlates strongly with terminal Clovis artifacts. Thus, observations of the platinum abundance in the YDB are consistent with expectations in every case. To refute this aspect of the YDIH, HEA must show that these predictions are not always satisfied. They have failed to do this.
6.3. HEA’s Section 5.5. Inconsistent dating of nanodiamond zones
HEA: Firestone et al. (2007) claim recovery of nanodiamonds from the YDB but present no data. Kennett et al. (2009a) first presented data claiming recovery of nanodiamonds in purported YDB zones. They discuss six sites across North America but provide plots (their figure 1) from only three sites (with no context on stratigraphy or depths) (Table 4). The Bull Creek site in Oklahoma is one of the three sites. Subsequent searches for nanodiamonds at Bull Creek were reported by Bement et al. (2014), Kinzie et al. (2014), and Sexton (2016). The research and discussions by the three groups is confusing and contradictory, however (see ENDNOTE 9 and Section 12.6).
HEA: Originally, Kennett et al. (2009a) claimed a nanodiamond peak of 100 ppb at 13.0 ± 1 cal. yr BP with ≈ 25 ppb of nanodiamonds at ≈ 10 cm above that level (plotted in their figure 1). Kennett et al. (2009a, figure 1 caption) write, “Stratigraphic profiles [showing no stratigraphy] on left show NDs only in the YDB” and hence identifies the YDB as spanning those two levels. Subsequently, Bement et al. (2014, table 1) reported orders of magnitude greater abundance of nanodiamonds at 307-312 cm below surface (cmbs) but that layer was undated.
Agreed, but Bement et al.’s (2014) units could be a typo.
HEA: Bement et al. (2014, table 1) identified the layer 298-307 cmbs as corresponding to the 100 ppb nanodiamond peak layer of Kennett et al. (2009a, figure 1) (Table 5) and also dated it to 11,070 ± 60 14C yr BP (~12,990 cal yr BP).
This is false and probably the source of HEA’s confusion, as already noted in relation to HEA’s Table 5 above. As previously mentioned, Bement et al. (2014) actually state,
Thus, Bement et al. (2014) actually associate the layer 307-312 (BC20) with Kennett et al.’s (2009) peak of 100 ppb.
HEA: However, Bement et al. (2014, table 1) attributes to Kennett et al. (2009a), without explanation, a different shaped nanodiamond peak than what is plotted in Kennett et al. (2009a, figure 1). The attributed peak has 100 ppb of nanodiamonds at 298-307 cmbs and 90 ppb at 307-312 cmbs.
Agreed. Bement et al. (2014) appear to have misplotted Kennet et al.’s (2009) data. They should have plotted 25 ppb at 298-307 cmbs and 100 ppb at 307-312 cmbs.
HEA: This attributed peak now overlaps with Bement et al.’s main peak position at 307-312 cmbs, whereas the peak plotted by Kennett et al. (2009a, figure 1) plots 0 ppb of nanodiamonds below the main 100 ppb peak and does not overlap with Bement et al.’s main peak.
This is false. HEA’s discussion is very confused and repeatedly intermixes correct and false statements. Bement et al. (2014) find a main peak of 190 ppm at 307-312 cmbs, and attribute this to the main peak of Kennett et al. (2009) of 100 ppb at the same depth.
HEA: Thus, Kennett et al. (2009a) illustrate a YDB spanning at least 10 cm at and above a date of ~13 cal ka BP …
HEA: … whereas Bement et al. (2014, table 1) identify a YDB spanning 14 cm at and below the ~13 cal ka BP date,
HEA: … with the orders of magnitude predominant peak in nanodiamonds clearly below that date (see further discussion in ENDNOTE 9).
Let’s be very clear about these two data sets. First, note that Bement et al. (2014) only sampled the YDB in the same “locality” as Kennett et al. (2009); they did not sample (to our knowledge) precisely the same sediment column. Therefore, deviations in measurements can occur due to small fluctuations in stratigraphy and concentrations between the sediment columns sampled. Taking this into account, let’s consider the data in question.
Kennett et al. (2009) identify the following nanodiamond concentrations near the YDB: 1) ~ 100 ppb corresponding to a radiocarbon date 13 ± 0.1 cal yr BP, and 2) ~ 25 ppb in a section ~ 10 cm above that. Each section is ~ 10 cm thick. No depth information is provided.
Bement et al. (2014) identify the following nanodiamond concentrations near the YDB: 1) 190 ppm in an undated section at 307-312 cm, and 2) 1.9 ppm in a section dated to 11,070 ± 60 C14 yr BP at 298-307 cm. They attribute these two sections to the same two sections of Kennett et al. (2009) above.
Thus, Kennett et al.’s (2009) peak of 100 ppb (dated at 11,090 ± 90 C14 yr BP by back-converting to a radiocarbon age using the same calibration curve) is associated with Bement et al.’s (2014) peak of 190 ppm (which is below a section dated to 11,070 ± 60 C14 yr BP).
Leaving aside the possible typo in Bement et al.’s (2014) units, there is no inconsistency here. While it is true that Bement et al. (2014) have incorrectly plotted the data in Kennett et al. (2009) and there is potentially a typo their units, the two data sets are consistent within error bounds (which are presumably quoted at 1 sd). The correct data is tabulated below.
HEA: Kennett et al. (2009a, 2015a), LeCompte et al. (2012), Bement et al. (2014), Wolbach et al. (2018b, 2020), West et al. (2020a), Powell (2020, 2022), and Sweatman (2021) all accept Bull Creek as evidence in support of the YDIH. However, as discussed in Section 12.6, the purported YDB nanodiamond concentration measurements are not credible. But even if the concentration measurements are accepted as accurate, as believed by YDIH proponents, the depth of the nanodiamond peak layers are clearly below the presumed YDB at Bull Creek and are inconsistent with the YDIH. ENDNOTE 9
As already stated, while there might be typo in the reported ppb/ppm units in Bement et al. (2014), as argued above there is nothing here that contradicts the YDIH. HEA seem to have misinterpreted the Bull Creek data, as indicated by their unexplained upward shifting of the Kennet et al. (2009) data in their Table 5 (shown above). Their ENDNOTE 9 continues to misinterpret this data. To be clear, we show Table 1 from Bement et al. (2014) in Figure 7 below. It confirms that HEA are mistaken.
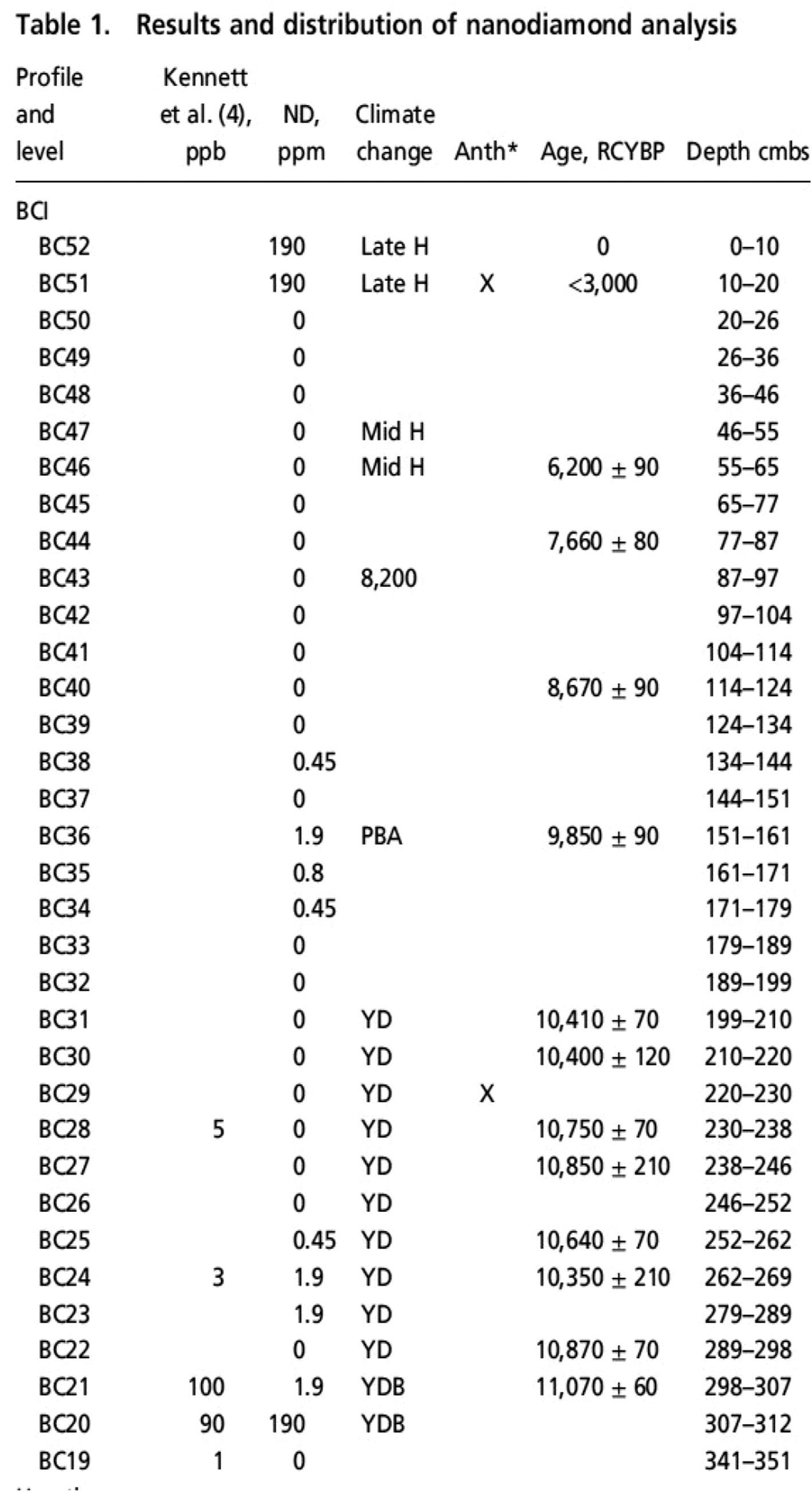
Table 1 from Bement et al. (2014) showing the correspondence between Bement et al.’s data and Kennett et al.’s (2009) data. Note, Kennet et al.’s (2009) data is mis-reported by Bement et al. (2014); the entry in BC21 should be 25 ppb while the entry in BC20 should be 100 ppb. This could be the source of HEA’s confusion.
HEA: Other claims regarding dating are equally curious, if not spurious. Kinzie et al. (2014) present data from 24 sites purported to show YDB nanodiamond spikes (but see also Table 3 and Section 12.6 regarding unreliability of those measurements). Eighteen of the claimed YDB zones are poorly dated, not dated, associated with a disconformity (Table 4), or from the Usselo soil, which formed through the Allerød and YD/GS-1 (Section 5.6). ENDNOTE 10
Kinzie et al. (2014) locate nanodiamond abundances at 24 sites precisely where they are expected based on either direct dating or indirect evidence such as terminal Clovis artifacts, just like for the platinum anomaly discussed earlier. HEA’s belief that this is a coincidence is preposterous. The Usselo Horizon is discussed next in HEA’s Section 5.6.
Corrected version of Table 5 of HEA shown in Figure 5.
Level | Depth (cm) | Kennett ND (ppb) | Bement ND (ppm) | C14 age (yr BP) |
---|---|---|---|---|
BC22 | 289-298 | 0 | 0 | 10,870 ± 70 |
BC21 | 298-307 | 25 | 1.9 | 11,070 ± 60 |
BC20 | 307-312 | 100 | 190 | 11,090 ± 90 |
BC19 | 341-351 | 0 | 0 | - |
6.4. HEA’s section 5.6. Logical Lapses in Dating and Interpreting Usselo and Finow Soils
HEA: Following on comments elsewhere (Holliday et al., 2020, p 87), YDIH proponents perpetuate logical lapses in the interpretation of radiocarbon dates and the dating of soils along with no understanding of soil forming processes in discussions of dates from the Usselo (Hijszeler, 1957) and Finow (Schlaak, 1993) soils (here simply referred to as the Usselo soil). These soils are charcoal-rich sands that act as distinct stratigraphic markers within widespread and genetically related layers of laterally continuous post-glacial eolian “coversand” sheets distributed across much of northwest and northcentral Europe (e.g., van Geel et al., 1989; Hoek 1997; Vandenberghe et al., 2013; Kaiser et al., 2009; van Hoesel et al., 2012, 2014; Andronikov et al., 2016a). The soils are not a “charcoal boundary layer” (contra Sweatman 2021, p 15).
An image of the Usselo charcoal-rich layer (horizon) is provided in Figure 8 above. It sits just above the YC I layer. There may be some misunderstanding of the labeling of the stratigraphy by some YDIH proponents, but the stratigraphy is clear in Figure 8.
HEA: Proponents of the YDIH (Firestone et al., 2007; Wittke et al., 2013b; Wolbach et al., 2018b, p. 190) argue that the charcoal in the soil is evidence of catastrophic biomass burning at the YDB or during the YD/GS-1 (depending on the author). Kennett et al. (2015a, figure 1) claim Usselo soils at Lingen (Germany) and Aalesterhut (The Netherlands) are of YD/GS-1 onset age based only on two dates from among scores of dates for Bayesian analyses (e.g., Hoek 1997; Kaiser et al., 2009). However, the geomorphologists and soil stratigraphers who were the principal investigators of the Usselo soil and know it best based on both field and Laboratory research…
HEA: …clearly demonstrate that the soil is just that: a zone of pedogenic weathering including accumulation of organic matter such as charcoal over time (van Geel et al., 1989; Hoek 1997; Kaiser et al., 2009; van Hoesel et al., 2012, 2013, 2014).
No arguments, other than spurious ones based on authority, are provided here to justify this assumption. Let’s be clear. There is no disagreement about how soils form or about the nature of the soils above or below the charcoal-rich layer. Instead, the debate concerns the charcoal-rich layer itself. The dating of this layer, established by independent groups to contain nanodiamonds and shocked quartz at two YDB sites (Tian et al. 2011; van Hoesel et al. 2015), is not inconsistent with the YDB, as we shall see below.
HEA: Kaiser et al. (2009, figure 8) illustrate the radiocarbon dating of 63 samples from the Usselo soil. The full range of dates spans almost 2000 years, but the bulk of the dates are from the Allerød interval (pre-YDB); far fewer date to the YDB or YD/GS-1. The dating is consistent with the field interpretation of prolonged pedogenesis before and during the YD/GS-1.
The focus of YDIH proponents is on this charcoal-rich layer. As argued by Sweatman (2021), radiocarbon dating of this charcoal-rich layer is not inconsistent with the YDB. The timespan of 2000 years is not problematic because most of these dates are based on single radiocarbon measurements and therefore their intrinsic radiocarbon measurement uncertainty is not a safe guide to their true sample age uncertainty. Only proper age-depth models at each site can provide a good estimate of the true age uncertainty at these sites. For example, when constructing their age-depth model, Gill et al. (2009) find radiocarbon dates scattered over 6000 years, suggesting individual radiocarbon dates can have an error of ~ 3000 years (at 2-sigma), which is at least an order of magnitude larger than their intrinsic radiocarbon measurement errors.
HEA: These data also directly contradict claims that half of the charcoal dates are at or near the YDB (Sweatman 2021, p 15) or that most of the Charcoal is in or on top of the upper soil zone and marks the YDB (Kinzie et al., 2014, p 477; Kennett et al., 2015a, p 4347, 4350; Wolbach et al., 2018b, figure A6) (Table 6). Based on the dating, including OSL ages for the eolian deposits above and below the Usselo soil, and the evidence for pedogenesis, van Hoesel et al. (2012, p 7651), van der Hammen and van Geel (2008, p. 360), and Kaiser et al. (2009) all reject the claim that the Usselo soil is a rapidly deposited YDB “event” layer.
If we consider the OSL data in Kaiser et al. (2009) first, every measurement recorded in their Table 3 (and plotted in their Figure 6B) is consistent with the YDIH. That is, all OSL measurements from below the charcoal-rich layer are consistent with an older age, all measurements from within the charcoal-rich layer are consistent with a YDB age, and all measurements from above the charcoal-rich layer are consistent with a younger age (all at 2-sigma).
If we consider Kaiser et al.’s (2009) radiocarbon measurements of the charcoal in this layer in their Table 2 (plotted in their Figure 6A), 3 of the measurements are from the same locality; Bledno, Poland. The standard error of these 3 measurements, 240 radiocarbon years, is much larger than the uncertainty in the 3 individual measurements (78, 80 and 70 radiocarbon years). Once again, this illustrates that the intrinsic radiocarbon measurement uncertainty is an unreliable guide to the true sample age uncertainty. Since Kaiser et al. (2009) examine 16 different localities, we would expect nearly all to be consistent with a YDB age at 2-sigma, and only one or two measurements to be between 2 and 3-sigma if the charcoal was produced by a synchronous event. Measurements beyond 4-sigma from a YDB age are unexpected. In fact, we find 11 out of 16 measurements are within 2-sigma of the age of the YDB. Bansin is within 3-sigma, leaving 4 sites outside of 3-sigma. Bachorze is only marginally outside of 3-sigma, which leaves just Wda 2 (Kaiser et al. (2009)) and Dybowo 1 and Obrowo 1 (Jankowski (2002)) appearing to be inconsistent with a synchronous event. However, we must remember that the intrinsic radiocarbon measurement uncertainty is an unreliable guide to the true sample age uncertainty. Since there is just one radiocarbon measurement from the Wda site (Wda 2), we suggest its measured uncertainty is unreliable, just like measurements from the 3 Bledno sites. In fact, its measured radiocarbon age and age uncertainty are very similar to the data from Bledno 2. Unfortunately, Jankowski (2002) does not appear to be available online. However, later work by Jankowski from the same locality (the Torun Basin, Poland) is available. In that work, Jankowski (2012) also favors a non-ET impact origin for the charcoal in the charcoal-rich Usselo/Finow layer, yet both radiocarbon measurements provided for that layer are consistent with a synchronous event of the same age as the YDB (11100 ± 270 C14 yr BP and 11100 ± 230 C14 yr BP). Therefore, the Usselo/Finow charcoal-rich layer is not inconsistent with synchroneity and the YDIH.
HEA: YDIH proponents nevertheless persist in using the Usselo soil as a YDB marker (Sweatman, 2021, p 12, 14, 15; Powell 2022, p 5). Sweatman (2021, p 12), for example, suggests that the soil represents “YDB sediment” based on no data and misunderstanding what a soil represents. He argues that the “conclusion that these sites are not synchronous should be considered inconclusive” even though the published field data and geochronology establish the Usselo as one of the best dated and stratigraphically consistent post-LGM terminal Pleistocene marker soils in Europe.
The use of single radiocarbon measurements at most Usselo/Finow Horizon sites is not consistent with the statement “… the Usselo as one of the best dated…marker soils in Europe”. Radiocarbon dating of this charcoal-rich layer is not inconsistent with a synchronous event and the YDIH, and HEA do not present or cite any evidence to contradict this position.
HEA: In his review of the YDIH, Sweatman (2021) includes a number of other critiques of the radiocarbon dating of the Usselo soil. The large body of consistent data generated by multiple investigators, which inconveniently contradict the YDIH, is offhandedly dismissed by an unsubstantiated remark that critics misunderstand “the nature of variance in the radiocarbon dating of sediments” (Sweatman, 2021 p 14).
HEA: The dating of the Usselo/Finow soil is based on scores of dates on individual fragments of charcoal, not sediments, from multiple sites. Sweatman (2021, p. 12, 15, 16, 17, 20) also criticizes reliance on single radiocarbon dates. Multiple samples for numerical age control are ideal, but in the early decades of numerical dating, not common.
This indicates that early conclusions formed about the Usselo/Finow Horizon were premature, as they were based mainly on individual radiocarbon measurements at each site. Proper age-depth models at each site should be used to estimate the true variance in the age of the charcoal horizon at each site. Until these measurements are made, the claim that the charcoal horizon is not consistent with the YDB cannot be made with any confidence. Indeed, it is remarkable that HEA strongly criticize the competent age-depth modeling of YDIH proponents which are often based on multiple radiocarbon measurements from each site and Bayesian statistical methods (e.g. Kennett et al., 2015a), but are content with mainly single radiocarbon measurements and outdated methodologies from most investigations of Usselo/Finow sites. This seems to be an inconsistent position.
In fact, in Kaiser et al. (2009) we read “In The Netherlands, 23 radiocarbon dates from various Usselo soil occurrences … cluster around 11000 14C yr BP (= 12900 cal. yr BP) (Hoek 1997).” Thus, this data appears to be consistent with a YDB age. Furthermore, Figure 8 from Kaiser et al. (2009) (shown below in Figure 9) confirms that 63 radiocarbon measurements on charcoal in the Usselo/Finow horizon also cluster around the YDB.
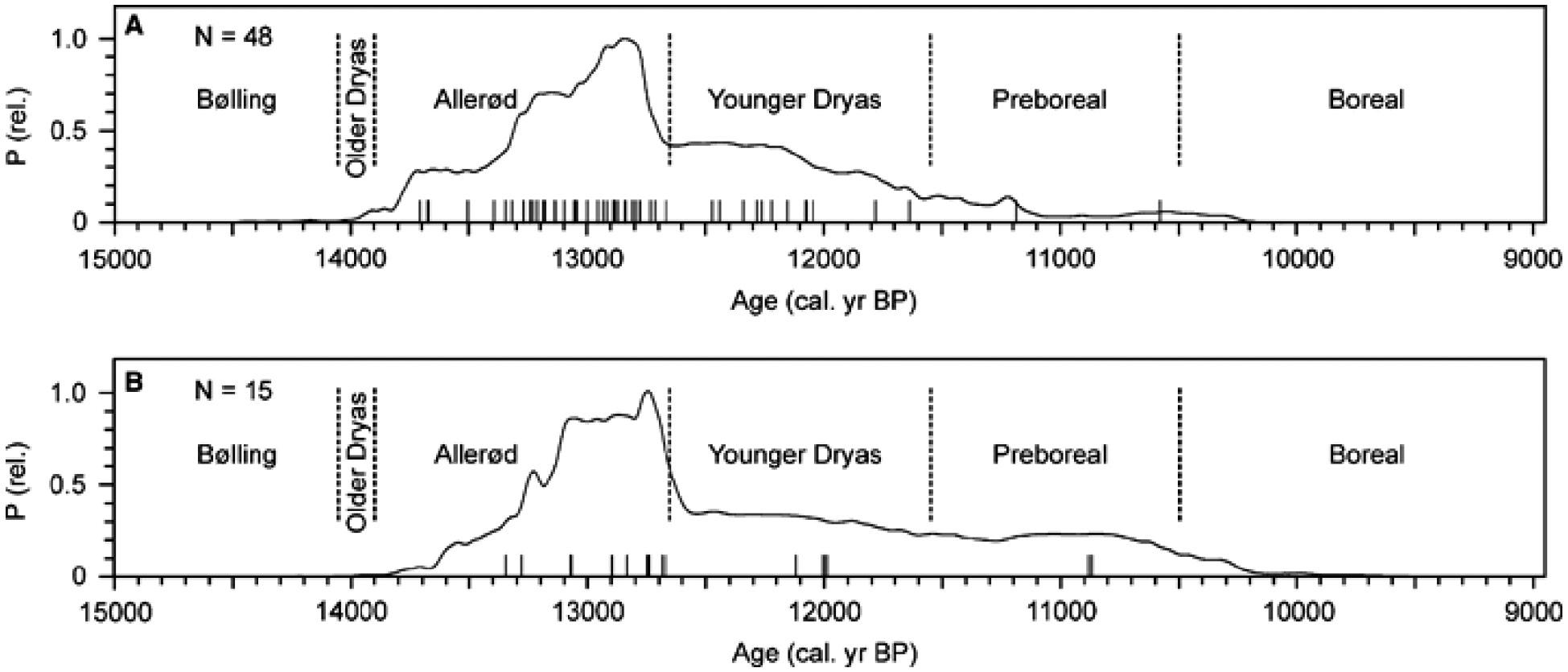
Figure 8 from Kaiser et al. (2009), shows that 48 radiocarbon ages from the Usselo horizon and 15 from the Finow horizon all cluster around the date of the YDB (~12,800 BP), with peaks in the summed probability distribution very close to a YDB age.
HEA: A single date is not by definition in error.
As shown above in relation to Gill et al (2009) and Meltzer et al. (2014), there is ample evidence that the intrinsic radiocarbon measurement error of a single measurement is not a reliable estimator for the true uncertainty in the age of a sample. This is also apparent from the Bledno site in Poland mentioned above. Thus, individual radiocarbon measurements at a site should be considered unreliable. This is why it is best practice to take multiple measurements from a site and construct competent age-depth models.
HEA: There are many examples of reliable single dates.
HEA: Indeed, Sweatman (2021, p2-3, 16) embraces the dating of the “black mat” by Haynes (2008) (Section 6) even though most of that dating is based on one or a few dates, and he expresses no concern over the issue of variance in that dating.
The issue is whether the Younger Dryas boundary at any site is clearly inconsistent with the date of the YD impact. So far, no YDB site has been found to be obviously inconsistent with the date of the YD impact.
The dating of the Usselo soil also raises an important point. Sweatman (2021, p 12) argues “the uncertainty in the age of YDB sediments is rarely captured by a single radiocarbon measurement at a specific site. Indeed, it is standard practice to take in the region of 10 measurements at any site to create proper age-depth models so that the true age uncertainty in a boundary layer can be reliably reported. Reliance on single measurements from any site is unwise, as we can expect such an approach to give the false impression of asynchronous local events for a synchronous widespread event across all sites.” This passage is rife with misleading inferences and a fundamental lack of understanding of the Usselo soil.
HEA provide no arguments or evidence to substantiate their claim that this passage is “rife with misleading inferences … of the Usselo soil”. In fact, the passage does not even mention the Usselo soil.
HEA: More broadly, this sweeping statement is a rather remarkable claim in support of the YDIH given that almost no site presented in the YDIH literature meets the requirement for 10 14C measurements for each YDB or black mat section.
This is misleading because it supposes that 10 measurements is a “requirement”. It is not. It is simply a preference. More measurements are better than fewer because they provide a better estimate of the true variance in the radiocarbon date of the samples. This is the principle of the Law of Large Numbers, a basic principle in statistics and science. A single measurement cannot give any indication of the true variance, by definition. Furthermore, the “10 14C measurements” mentioned by Sweatman (2021) were in the context of constructing an age-depth model, which models the depositional environment over time throughout an entire stratigraphic section and not just within a single horizon or boundary layer like the YDB.
HEA: This argument could be used to consider the notion of the YDIH equally inconclusive. He further argues (p 15) that in dating the Usselo soil “only single measurements were made at each site. Proper age-depth models that intersect the boundary were not generated for any of them, leaving open the possibility that the sites are synchronous and the dispersion in dates they found was due to natural processes.” This is another unsupported assertion with no factual basis.
As already argued, single measurements at a site are unreliable in principle. This is demonstrated by the radiocarbon data of Gill et al. (2009), but it is also clear in the Bledno data in Kaiser et al. (2009). HEA provide no evidence against this position.
HEA: Again, similar to Kennett et al. (2015a), an assumption is made that because it could represent the YDB, it therefore must be the YDB.
This is false for all the reasons given above and no YDIH proponent takes this position. It is an invention of HEA.
HEA: More to the point, an impact, representing a moment in time (similar to a volcanic eruption such as the Laacher See; Baales et al., 2002, and Section 5.8) produces radiometric dates that vary around a mean. The Usselo soil, in contrast, produced many non-overlapping radiocarbon dates spanning 1400 14C years because it is a soil. There is no possibility that the sites are synchronous.
The issue is whether any of these non-overlapping dates for the charcoal-rich layer spanning 1400 radiocarbon years are inconsistent with the date of the YD impact. Given that most of these measurements correspond to single radiocarbon measurements their true age uncertainty is unknown in principle. Thus, it cannot be concluded with any confidence that any of the single dates for the charcoal at these sites is clearly inconsistent with the YD impact as HEA claim. See Gill et al. (2009) in our Section 4 which demonstrates that the true uncertainty (i.e. scatter or variance) in radiocarbon measurements can far exceed that of the radiocarbon measurement itself, which is why multiple measurements leading to proper age-depth models are preferred.
HEA: The Usselo soil does not represent a moment in time.
As we have just argued, it is not possible to claim this with any confidence with the present radiocarbon data set for the charcoal in this charcoal-rich layer (the Usselo/Finow horizon).
HEA: Sweatman (2021, p 15) also asserts “the precise boundary layer at each site corresponding to the depth of geochemical markers, rather than charcoal which is not diagnostic for the impact event, was not determined for any site studied, and therefore it is not possible to know if any charcoal samples were taken directly from the Younger Dryas boundary.” That is another example of circular reasoning, however.
HEA do not explain how this is circular reasoning. In fact, it is simply a statement about a prediction of the YDIH which is that YDB at each site is defined by impact proxies. HEA appear to lack the logic required to properly test the YDIH.
HEA: Sweatman claims the geochemical signatures define the YDB, while at the same time they are interpreted as impact markers largely because they occur at the YDB and are synchronous with the YD/GS-1 onset, which is claimed synchronous with the megafauna extinctions.
As has been stated many times already, to be considered a YDB site, impact markers must be present with an age not inconsistent with the suggested age of the YD impact or be strongly correlated with youngest Clovis artefacts. This is perfectly clear. Moreover, HEA imply that YDIH proponents consider any material to be an impact marker if it lies within the YDB. This is absurd. Materials are considered to be impact markers because of their physical and chemical properties, not for any other reason. These material properties are investigated by highly discriminative processes using state-of-the-art instruments.
HEA: While we agree that charcoal is not an impact marker (see Section 9.3), YDIH proponents repeatedly claim that it is produced in great quantity by the YDB impact. For example, “Wildfire … at the Younger Dryas boundary” is the title of Kennett et al. (2008a) and the titles of both Wolbach et al. (2018a, b) begin “Extraordinary biomass-burning … triggered by Younger Dryas cosmic impact.” Sweatman (2021, p 12) earlier cites these and other papers purporting peaks in charcoal at the YDB. Therefore, charcoal is certainly an appropriate material to use for dating the soil and the dates clearly show that it is both older than and younger than the YDB.
We agree that charcoal is an appropriate material for dating the charcoal-rich layer. Nevertheless, the YDB is technically defined by impact proxies, not charcoal.
HEA: Confusingly, some YDIH proponents explicitly claim the black mat lies directly above the YDB, while others claim it is the YDB (see Section 6).
There should be no confusion when one realizes the YD black mat is not the same everywhere. However, the YD black mat is generally a good guide to the position of the YDB where it exists. In some places, such as Mt Viso in the European Alps (Mahaney et al., 2023), there is no clear distinction between the YD black mat and the YDB, while in other cases, the YD black mat is rich in charcoal (e.g., the Usselo and Finow horizons, Europe), but these characteristics are not true generally.
The YD boundary, however, is always defined by the YD impact proxies, and this is often, but not always, found at or near the base of the YD black mat.
HEA: Sweatman (2021, p. 16) on the other hand apparently alludes to both, claiming the base of the black mat (and the Usselo Soil) is the YDB and the remaining majority formed over the YD/GS-1. The Usselo Soil cannot (and does not) represent both the YDB impact and the YD/GS-1.
This statement about the “Ussello soil” is unclear. As already stated, radiocarbon dating of the charcoal-rich Usselo/Finow layer is not inconsistent with the date of the YD impact. Technically, however, the YDB is defined by the location of impact proxies. This is clear.
HEA: The obvious conclusion based on all geologic and pedologic data, including the dating of deposits above and below the charcoal-rich soil horizon is that the Usselo soil reflects fires (along with pedogenesis) spanning at least ~1400 14C years, largely in the Allerød but continuing into the YD/GS-1 (i.e., across the YDB).
This statement cannot be made with any confidence. As already argued, the radiocarbon data for the Usselo charcoal-rich layer in the soil is not inconsistent with the YDB. Impact proxies are found in the charcoal layer at a few sites, and charcoal is an expected product of the YD impact. Thus, it is sensible to presume that the charcoal layer is a useful guide to the position of the YDB. Only proper age-depth models at each site along with the detection of geochemical impact proxies can show if this assumption is correct.
HEA: A bigger issue among YDIH proponents is a fundamental misunderstanding of the nature of pedogenesis, which is a time transgressive process on stable or quasi-stable landscapes. ENDNOTE 8 “[T]here is no need to invoke an extraterrestrial cause to explain the charcoal in the fossilized soils” van der Hammen and van Geel (2008, p 359).
6.5. HEA’s Section 5.7. Improved dating of Clovis sites and Clovis archaeology
HEA: Clovis is a term given to the oldest well-dated, widespread, and recognizable archaeological technocomplex in North America (Haynes, 2002; Smallwood and Jennings, 2015; Meltzer, 2021). Proponents of the YDIH use their perceived connection between the disappearance of the Clovis lithic tool style and the onset of the YD/GS-1 stadial at ~12.9 ka BP as evidence for an environmental catastrophe (Sections 1, 3.1 and 3.2) (e.g., Firestone et al., 2006, 2007; Anderson et al., 2011; Wolbach et al., 2018b).
This is misleading as the date of the “YD/GS-1 stadial at 12.9 BP” is provided by HEA and is not a date YDIH proponents adopt. Moreover, HEA are incorrect. YDIH proponents do not consider the rapid disappearance of the Clovis toolkit as evidence of an ET impact. It is, however, consistent with an ET impact.
HEA: Powell (2020) repeats the notion of a non-existent mystery regarding the disappearance of Clovis archaeology (Section 3.1). Subsequently, Powell (2022) is quite emphatic on this point. He refers to “the fall of Clovis” (p 35) and claims (p 36) “just at its prime [~13 ka], Clovis suddenly fell” and “No Clovis artifacts have ever been found in place above the YD” (presumably referring to the YDB). These claims are false.
The most precise dating of the “fall of Clovis” is provided by Waters et al. (2020). Powell’s (2022) comments above are consistent with their conclusions (see below) which are consistent with the YDIH.
HEA: The YDC is a time interval spanning ≈ 1200 calendar years. A broad variety of artifacts styles appeared and disappeared during and after the span of the YD/GS-1 in North America. For example, most of the data used by Anderson et al. (2011) are undated (and undatable) artifacts found on the surface. Radiocarbon dating of Clovis sites (below) shows that Clovis persisted beyond the YDB.
This is, once again, misleading. Clovis artifacts might have persisted for perhaps a century or two after the YD onset, but this is uncertain and does not contradict the YDIH. What is clear, however, is that no Clovis artifacts have ever been found above the YDB. This suggests that Clovis people endured a catastrophe that soon led to their demise.
HEA: Powell (2022, p 36) offers other unsubstantiated and factually incorrect claims regarding Clovis archaeology. “At the Topper site, LeCompte et al. [2012] found impact microspherules touching Clovis artifacts, but no microspherules below the artifact layer.” He apparently is unaware that the archaeologist who excavated the Clovis and younger components at Topper documents the mixing of the assemblages (Miller, 2010). The context of spherules in a single sample column is meaningless.
The finding of exotic microspherules in contact with Clovis artifacts is a prediction of the YDIH. It therefore defies logic to claim, as HEA do, that when a prediction of the YDIH is confirmed that this somehow counts against the YDIH.
HEA: Powell (2022, p 36) further claims “In the Southeastern US, near the onset of the YD, the Clovis suddenly abandoned a dozen Paleo-Indian chert quarries” with no citation. Topper was a quarry but also a primary habitation. No Clovis quarries with firm age control are reported.
Again, these observations are not inconsistent with the YDIH and cannot be used as evidence against the YDIH. HEA’s view defies logic once more. Powell was referring to the work of Anderson et al. (2011).
HEA: More generally he notes “In the eastern US, Clovis artifacts have been found from Maine to Florida, where average yearly temperatures differ by much more than the ~10°C change at the beginning of the YD. Could such a relatively small temperature change, even one that occurred rapidly, by itself have destroyed such a well-adjusted and widespread culture?” Leaving aside the bizarre comment about a “well-adjusted” culture, the quote reveals a misunderstanding of the difference between the annual cycle of temperature and changes in long-term mean global annual temperatures. In any case, no data are provided to support these assertions.
No arguments or evidence are presented by HEA to refute the YDIH here either. Considering their claim is to refute the YDIH, they are required to present dispositive evidence that contradicts the YDIH, and so far they have failed to do so.
HEA: But Fastovich et al. (2020) and Griggs et al. (2022) show that environmental conditions across eastern North America before, during, and after the YDC varied significantly in space and time. But the point is essentially moot. Clovis populations survived across North America in highly varied and changing environments from before and into the YDC (e.g., Haynes, 2002; Smallwood and Jennings, 2015).
We agree, the point is moot. The issue is whether the YD impact significantly affected Clovis populations. Since no Clovis artifacts have ever been found above the YDB, this suggests they were. Recall that Haynes (2008) states that “no post-Clovis Paleoindian artifacts have ever been found in situ stratigraphically below [the YD black mat].” The lower boundary of this black mat often marks the position of the YDB. He further states that “The YD black mat covers the Clovis age landscape on which the last skeletal remnants of Rancholabrean megafauna occur” and although the “ages of the youngest Clovis sites overlap with those of the oldest Folsom sites…the stratigraphic separation is clear…The megafaunal extinction and the Clovis-Folsom transition appear to have occurred in <100 years, perhaps much less.” He concludes, “A remarkable major perturbation occurred at [the YDB] that needs to be explained.”
HEA: Radiocarbon dating shows that there is no correlation between the YDB and the end of the Clovis archaeological style.
HEA: The work of Waters and Stafford (2007) was accepted as a standard for the dating of the Clovis occupation of North America by the YDIH proponents (e.g., Firestone et al., 2010a; Wittke et al., 2013a; Kennett et al., 2015a) although their dating did not quite support the YDIH claims. Waters and Stafford (2007) suggest that Clovis occupied a narrow time window between ~13.0 ka and ~ 12.6 ka. That age range was revised/updated and now indicates that Clovis largely post-dates 12.9 ka by up to several centuries (Waters et al., 2020).
This is misleading. Age comparisons should be made with raw uncalibrated radiocarbon data. As we shall see, the uncalibrated data provided by Waters et al. (2020) is consistent with the YDIH.
HEA: That paper (published before Sweatman, 2021, was submitted) …
HEA: … proposes a maximum calibrated age range for Clovis of ~13,050 to ~12,750 cal yr BP. Their fig. 2 shows that most of their dated sites post-date the YDB. Only one is clearly older.
Figure 2 from Waters et al. (2020) shown above in Figure 10 clearly shows that they infer that a megafaunal extinction coincides with the end of Clovis, within dating uncertainties. It is important to note that the date of the Younger Dryas onset depicted in this Figure is not the one adopted by proponents of the YDIH and is shown without any uncertainty. In fact, Waters et al. (2020) conclude;
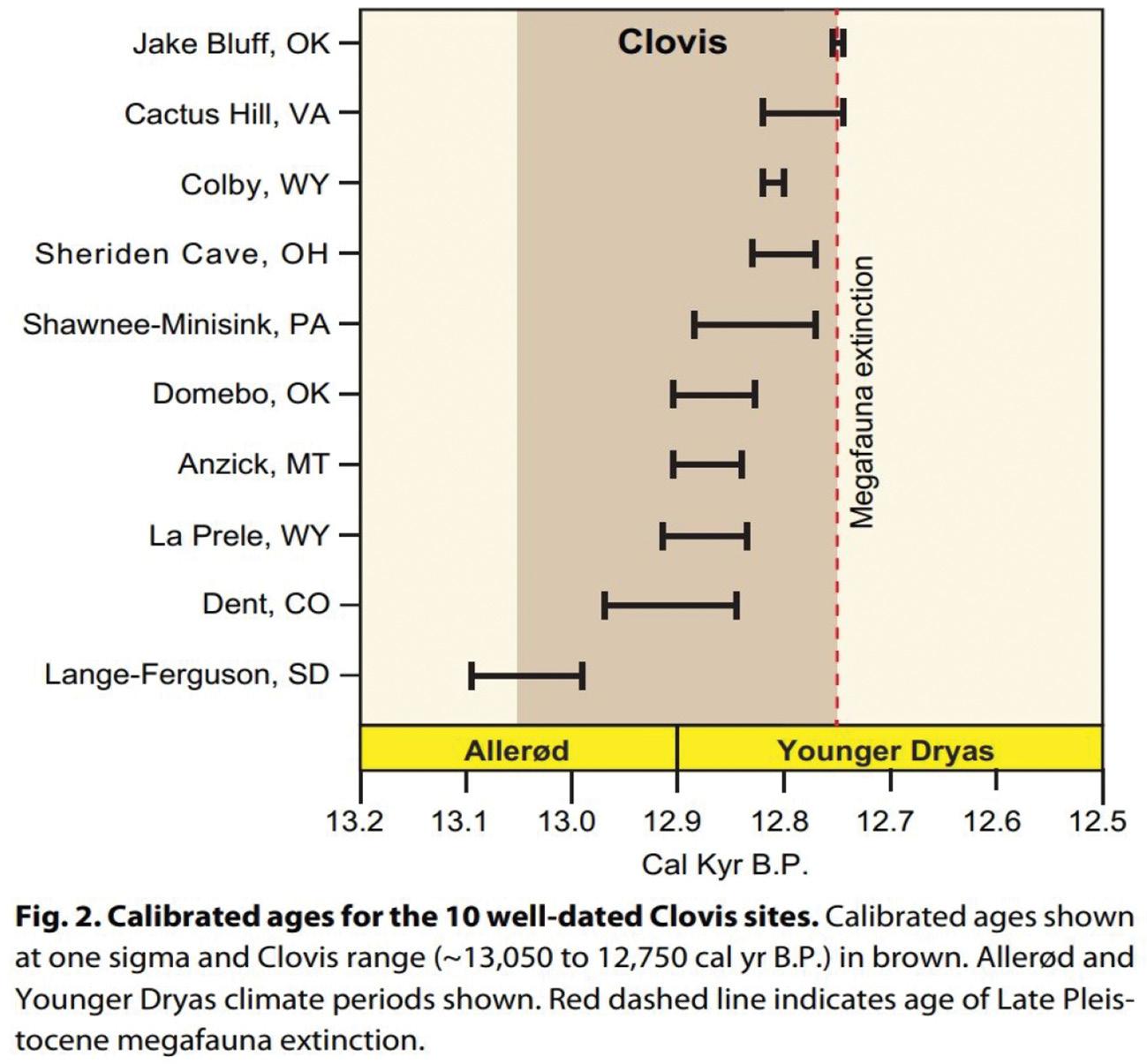
Figure 2 from Waters et al. (2020) shows the correlation between Clovis archaeology, a megafaunal extinction and the onset of the Younger Dryas.
“Clovis — the technology — abruptly ends at ~12,750 cal yr B.P. and coincides temporally with the beginning of the Younger Dryas cooling event and palaeontologically, with the extinction of Mammut, Mammuthus, and Cuvieronius. Archaeologically, Clovis terminates immediately before the emergence of Folsom technology on the Plains (27, 46) and the eastern Fluted Point Tradition in the eastern United States (47). In contrast, the production of stemmed points in the western United States continues after Clovis ends (48).”
Thus, the view of Waters et al. (2020) is that the end of Clovis, a megafaunal extinction event and the YD onset are essentially synchronous within dating uncertainty.
Figure 2 from Waters et al. (2020) shown in Figure 10 above compares the onset of the Younger Dryas, a megafaunal extinction and the end of Clovis on a calibrated age timescale. However, this comparison is best made with uncalibrated ages to avoid confounding the issue with calibration uncertainty. Taking the estimated age of the YDB from Kennett et al. (2015a) of 12,735-12,835 ± 50 cal BP (2-sigma), we can back-calculate the radiocarbon age using the same 2013 calibration curve to find the age of the YDB is 10,965 ± 1 C14 yr BP. Note, the uncertainty in the calibrated age is almost entirely due to the calibration curve. If we compare this age with the average age for each Clovis group investigated by Waters et al. (2020), we find that 8 out of 10 groups are consistent with an older age than the YDB (at 2-sigma). The only groups with apparently younger average ages are those at Jake Bluff (10,820 ± 10 C14 yr BP) and Cactus Hill (10,860 ± 20 C14 yr BP).
Therefore, if we take these radiocarbon results at face value, they are consistent with the YDIH which predicts that the YD impact significantly affected the Clovis culture. We emphasize that the YDIH does not predict that the impact wiped out Clovis entirely in an instant.
However, Waters et al. (2020) do not compare the archaeology or paleontology to the YDB because they do not locate the YDB at any site. Therefore, there remains some doubt about their results in terms of synchroneity with the YD impact. As we have emphasized several times, the archaeology, paleontology and paleoenvironmental indicators should always be related to the position of the YDB when assessing the YDIH. It remains the case that no Clovis artifacts have ever been found above the YDB.
HEA: Further, Buchanan et al. (2022), using recent dating of Folsom archaeology (Buchanan et al., 2021) along with the work of Waters et al. (2020) demonstrate an overlap of the two artifact traditions by as much as 200 years, discrediting the notion of an abrupt cultural termination at the YDB (and the notion of some sort of occupation hiatus after the Clovis occupation, Section 1) (see also Barlow and Miller, 2022).
Recall, Haynes states that although the “ages of the youngest Clovis sites overlap with those of the oldest Folsom sites…the stratigraphic separation is clear…The megafaunal extinction and the Clovis-Folsom transition appear to have occurred in <100 years, perhaps much less.”
Furthermore, HEA’s statement also appears to be at odds with the view of Waters et al. (2020) who state that, “Archaeologically, Clovis terminates immediately before the emergence of Folsom technology on the Plains (27, 46) and the eastern Fluted Point Tradition in the eastern United States (47).”
This illustrates the problem with HEA’s approach which values radiocarbon dates more highly than stratigraphy. In any case, the point is moot since the YDIH makes no predictions about other cultures. Our main concern in this section is instead the extent to which Clovis artifacts appear above the YDB. So far, none have been found.
HEA: One notable example of flawed dating ignored by the YDIH proponents is from the Gainey archaeological site in Michigan (Table 4). This badly mixed Clovis site was repeatedly presented as a key locality supporting the YDIH (Firestone et al., 2007; Bunch et al., 2012; LeCompte et al., 2012; Wittke et al., 2013a; Kennett et al., 2015a, 2015b) although the absence of intact context at the site was emphatically stated by the archaeologists that investigated it and repeatedly stated by YDIH critics (Holliday and Meltzer, 2010; Boslough et al., 2012; Holliday et al., 2014; Meltzer et al., 2014). Significantly, the site is the only YDIH locality where purported impact indicators are directly dated, yielding ages of ~200 and -135 14C yr BP (Table 4). They are clearly not YDB age and one, from R. Firestone, must be from a modern sample that included “bomb carbon” (from atmospheric testing of nuclear weapons) which yields radiocarbon dates from the future, a well-known problem in radiocarbon dating of young samples (Taylor and Bar-Yosef, 2014, p 23). Despite the obvious damning data on the context and age of the site, some years after it was published, YDIH proponents continued to maintain that it is a YDB site (Kennett et al., 2015a, SI pS34; Powell, 2022).
6.6. HEA’s Section 5.8. Radiocarbon simulations of the YDB
HEA: Inter-site variability in radiocarbon dates on purported impact proxies has remained problematic for the YDIH, suggesting that those layers were deposited asynchronously (Holliday et al., 2014; Meltzer et al., 2014).
We already showed above that Meltzer et al. (2014), and therefore Holliday et al. (2014), is fundamentally flawed. The statistical argument presented above demonstrates the YDB sites are very likely synchronous, or highly correlated over a short time-span.
HEA: However, YDIH proponents continue to argue that the layers were deposited synchronously and have generally supported this argument by citing Kennett et al. (2015a; but see Boslough et al., 2015; Holliday, 2015). Using a OxCal, with a Bayesian age model implementation, Kennett et al. (2015a) estimate upper and lower chronological boundaries for a hypothetical temporal phase containing supposed impact-indicators from 23 sites in addition to seven paleoclimatic proxy markers of the YD/GS-1 onset. Kennett et al. (2015a, pE4352) estimate a temporal difference between the start and end of the proxy phase somewhere within 0–130 years (95% probability interval), concluding that synchronous deposition of all 23 layers is plausible since the range of possible years includes zero. Unfortunately, Kennett et al. (2015a) neither plot nor describe the mean, median, or mode of this interval, so it is difficult to assess which temporal distances are most probable–while zero years may be plausible, this interval is also consistent with distances exceeding a century.
Agreed, 0 years is plausible. Again, the statistical argument above demonstrates the YDB sites are very likely synchronous, or at least highly correlated over a short time-span.
HEA: Further, the assumptions and decisions involved in the creation of this phase model render its inferences problematic. Given the many parameters and assumptions required to model the 23 site chronologies, it is unclear to what degree this 0–130-year estimate is contingent on modeling decisions. These decisions include the placement of stratigraphic breaks, the inclusion/exclusion of horizontally disparate samples, chronometric hygiene protocols, as well as the distributions, types, and prior parameter values of site-specific age models and of sample-specific outlier models. Additionally, the probability distributions of the start and end of the proxy phase result from the choice to include the 23 modeled YDB ages in a single temporal phase, which itself has multiple possible distributions and prior parameter values that must be specified by the user. In combination, these decisions compound potential problems stemming from assumptions at different modeling levels. Despite these issues, subsequent works that favor the YDIH cite Kennett et al.’s (2015a) model as confirmation that the proxy layers represent one event (e.g., Israde-Alcántara et al., 2012; Moore et al., 2017), while it remains, at best, only plausibly consistent with synchroneity.
Agreed, Kennett et al. (2015a) only shows plausibility for synchroneity. Nevertheless, it is shown explicitly above to be almost certainly correct. Essentially, the probability of the null hypothesis model (they are unrelated impacts and therefore not synchronous) is shown to be vanishingly-small.
HEA: In addition to the previously discussed evidence contradicting the assertion that the purported proxy layers were deposited by a single event (Section 5.0), simulations published by Jorgeson et al. (2020) illustrate that the impact proxy radiocarbon dates used by Kennett et al. (2015a) are far more dispersed than expected for a synchronous event.
Sweatman (2021) already critiqued Jorgeson et al. (2020) and showed that that their models are not adequate to support their conclusions with any confidence.
HEA: Jorgeson et al.’s (2020) simulations iteratively sampled radiocarbon ages from a hypothetical synchronous event, accounting for uncertainty in the radiocarbon calibration curve, laboratory measurement error, and old wood effects. The authors compare age dispersion in the simulated samples to the age dispersion of the observed YDB radiocarbon sample dataset and to the age dispersion in observed radiocarbon samples of a known synchronous event, the Laacher See volcanic eruption in Germany. The YDB radiocarbon dataset shows far more age dispersion than the simulations, while the Laacher See volcanic eruption radiocarbon dataset displays age dispersion similar to the simulations.
Again, Sweatman (2021) showed that Jorgeson et al.’s (2020) arguments are flawed. Jorgeson et al. (2022) responded, but Sweatman (2022) showed their response was also flawed. Like HEA and Meltzer et al. (2014), Jorgeson et al. (2020, 2022) do not properly understand the limitations of radiocarbon age modeling. Indeed, the key problem for Jorgeson et al. (2020, 2022) is stated by Sweatman (2022) to be “an unsupportable confidence in modeling the radiocarbon data from the Younger Dryas boundary”. In other words, a model is only as good as its underlying assumptions. Thus, the discrepancy between their model and YDB radiocarbon data cannot be attributed solely to the assumption of synchroneity in the YDB radiocarbon data with any confidence. In fact, given the statistical argument above about the YDB synchroneity, it is clear the problem instead lies with the assumptions that define Jorgeson et al.’s (2020) model.
Indeed, it is clear that their modeling approach is fundamentally flawed from the outset. As shown in our Section 4, since the intrinsic radiocarbon measurement error is an unreliable estimate for the true sample age uncertainty, individual radiocarbon measurements should be viewed with caution. Instead, multiple radiocarbon measurements from the same or neighboring sections should be used to obtain a more accurate estimate of the true sample age uncertainty. However, Jorgeson et al. (2020) take the opposite approach, favoring individual measurements from the YDB at different sites. This is inherently unreliable.
In fact, they use only 19 radiocarbon measurements from the boundary layer of the Laacher See eruption, but 30 radiocarbon measurements from the Younger Dryas boundary. In every case, the intrinsic radiocarbon measurement uncertainty is used as a proxy for the true sample age uncertainty. There is, therefore, already a much higher chance that consistency issues will be found with the YDB data set, even if both data sets represent synchronous events.
There is also another fundamental inequity in this comparison of radiocarbon data sets. All 19 dates from the LSE boundary used in the model come from six sites within a ~30 km radius in Germany, while the 30 dates from the YDB are from 23 sites dispersed over more than 13,000 km. This simple fact on its own could account for much of the variation between the models; it is simply a function of inter-site variability and the number of sites examined. Indeed, region-specific radiocarbon calibration curves for terrestrial material have been identified as a potential future development (Reimer 2021), made necessary because the distribution of atmospheric radiocarbon varies by region, and especially by hemisphere.
HEA: YDIH proponents - mainly Sweatman (2021, 2022; see also Powell, 2020) - raise four objections to Jorgeson et al.’s simulations, but each lacks merit. The objections involve old wood effects (see also Section 12.4) for the Arlington Canyon radiocarbon dates, the effects of catastrophic geomorphic processes on the integrity of radiocarbon samples, inadequate chronological modeling, and a failure to address the supposed geochemical evidence for the hypothesis.
These four issues are not addressed adequately by Jorgeson et al. (2022) or HEA, as we shall see. Two more issues were added above, specifically;
Jorgeson et al.’s (2020) approach, which uses the intrinsic radiocarbon measurement uncertainty as a proxy for true sample age uncertainty, is inherently unreliable. It will favor sites where this correspondence is stronger, but this correlation is unrelated to synchroneity. Instead, multiple measurements from each site should be preferred, which can be used to construct competent age-depth models.
The data sets are not comparable in terms of the number of samples, sites, or their geographical range, which could have a significant influence on their apparent consistency through inter-site variability and regional differences in the distribution of atmospheric radiocarbon.
HEA: Concerning Arlington Canyon, Sweatman (2021, 2022) argues that the old-wood offsets used in Jorgeson et al.’s (2020) simulations are not sufficient to account for pine species at the site, which can live up to 1000 years. As such, Sweatman argues that a synchronous event should produce radiocarbon samples more dispersed than those simulated by Jorgeson et al., as larger old-wood offsets would generate more temporal variability. As reported in Kennett et al. (2008a), 7 of 11 radiocarbon samples from Arlington Canyon are wood charcoal. These wood samples consistently predate Kennett et al.’s (2015a) modeled YDB age by only ~0–450 14C yrs, an offset that is well accommodated by the simulated old-wood offsets (Jorgeson et al., 2022). As such, the high dispersion in YDB radiocarbon ages is not explainable in terms of the Arlington Canyon samples alone.
This is obviously false. The largest decay constant used by Jorgeson et al. (2020) in their exponential old wood model is 100 years, which is clearly inadequate for modeling a small sample with such high ages of 450 years.
HEA: The remaining four (of 11) Arlington Canyon radiocarbon samples comprise an unspecified charcoal, a “carbon spher[ule]”, a “carbon elongate”, and a “glassy carbon” sample. Supporters of the YDIH claim that carbon spherules, carbon elongates, and glassy carbon are remnants of burned tree sap (Israde-Alcántara et al., 2012, p E745; LeCompte et al., 2018, p 169; Wolbach et al., 2018b, p S27, 2020, p 99; but see Scott et al., 2010, 2017 and Sections 9.3 and 12.4), and that these Arlington Canyon samples were produced by biomass burning in the wake of the impact event (Kennett et al., 2008a).
YDIH proponents claim that charcoal samples in the YDB that contain impact proxies were produced by the YD impact. The YDIH makes no claims about samples that do not, or are not known to, contain impact proxies. Such samples could have been produced by non-impact wildfires. Since the samples used for dating Arlington Canyon are not known to contain impact proxies, HEA’s argument can be disregarded.
HEA: The spherule dates to 11,440 ± 90 14C yr BP, corresponding to a calibrated 95% interval of 13,458–13,163 yr BP (UCIAMS-36961; Kennett et al., 2008a), well prior to the proposed impact.
HEA: The carbon elongates and glassy carbon samples also have similar dates of 11,110 ± 35 and 11,185 ± 30 14C yr BP (UCIAMS-36962 and UCIAMS-36960; Kennett et al., 2008a), corresponding to calibrated 95% intervals of 13,100–12,924 and 13,162–13,085 yr BP, respectively. Like the wood samples, these samples are consistently older than the proposed YDB age. Unlike the wood samples, burned tree sap is not subject to old wood effects.
Agreed, but this does not refute the YDIH or the dating of Arlington Canyon because these samples were not examined for impact proxies. They may well be from earlier wildfires.
HEA: Kennett et al. (2015a) rely on treating these three specimen types as wood charcoal with potentially large age offsets, incorrectly allowing for a younger age for claimed impact indicators for Arlington Canyon consistent with the YDIH.
It is true that glassy carbon, carbon elongates and carbon spherules with large age offsets could have been excluded from the dataset. However, using OxCal, Kennett et al. (2015a) were able to include them anyway using an old wood model. Since it is not known whether they include any impact proxies, this is correct procedure. This is because if they were discarded it would likely make no significant difference to the date of the YDB. Indeed, HEA provide no evidence that excluding them would make any significant difference to the date of the YDB at that site.
HEA: If the Arlington Canyon layer dates to Kennett et al.’s (2015a) proposed YDB age, and if the carbon spherules/elongates as well as glassy carbon are wildfire products (as YDIH proponents claim), then those from earlier wildfires were mixed into that layer.
Agreed. This is very likely considering that Arlington Canyon is a ravine with frequent mixing of sediments due to turbulent high flow rate events.
HEA: In that case the carbon spherule/elongate and glassy carbon concentration profiles that have been published in support of the YDIH cannot be used the test the YDIH. This is because those specimens are not impact markers, thus in order to correlate any particular carbon spherule/elongate or glassy carbon to a possible YDB impact event (and to the same specific wildfire), it is then necessary to date that particle to the YDB. To test the YDIH, it is then necessary to radiocarbon date each and every carbon spherule/elongate and glassy carbon counted through the sediment profile to construct a meaningful concentration profile based on age-correlated abundances. This clearly has not been performed.
None of the samples used for dating at Arlington Canyon were also examined for impact proxies. Some of the samples used for dating may well have been produced by earlier wildfires and could have been excluded from the dataset, but the dating model was able to incorporate them using an old wood model. HEA provide no evidence that their exclusion would make any significant difference.
HEA: Given the current evidence, the parsimonious interpretation of the Arlington Canyon “proxy layer” is that it predates the hypothesized event, consistent with Jorgeson et al.’s (2020) simulations.
Given the impact proxies present in the Arlington Canyon (AC) YDB layer, based on the above statistical argument the most parsimonious explanation is that it is of YD impact age, and Jorgeson et al.’s (2020) model is inadequate.
HEA: If the samples from Arlington Canyon indeed attest to an interval of increased wildfire, there is an alternative hypothesis. The calibrated ages of the tree samples are consistent with sharp increase in Greenland δ18O values between the cooler GI-1b (the IACP, see Sections 3.3 and 9.2) and the warmer GI-1a interval (Rasmussen et al., 2014). At that time, Santa Barbara Basin ocean-surface conditions, which apparently vary synchronously with Greenland climate (Hendy et al., 2002), would have abruptly changed from cool to warm (and from cooler and drier to warmer and wetter conditions on adjacent land areas). The samples could well be the product of wildfire favored by that climate change and would not require an exotic explanation.
Agreed, but since these samples used for dating were not examined for impact proxies, this is irrelevant.
HEA: Sweatman (2022, p 3) also contends that inconsistent radiocarbon samples from Murray Springs and Big Eddy should have been discarded from the simulations. Jorgeson et al. (2020) consider the exclusion of questionable dates from Murray Springs and Big Eddy in their supplemental simulations - exclusion of these dates does not affect the main conclusions drawn from the simulations.
HEA fail to note Sweatman’s (2022) response that “… if any of their modeled scenarios do not simultaneously account accurately for the old wood effect at Arlington Canyon, or for the SOM effect at Murray Springs, or for the anomalous data point at Big Eddy, they will likely obtain a negative result. In fact, they do not consider any scenario that allows simultaneously for all three effects.” Thus, it is likely their model is inadequate.
HEA: In the second objection, YDIH proponents blame catastrophic geomorphic processes for high variability in radiocarbon ages between layers containing purported impact proxies. Sweatman (2021) initially argues that the radiocarbon record is consistent with synchronous deposition. Yet, one year later, Sweatman (2022, p 1), in response to Jorgeson et al.’s simulations, argues that high dispersion in radiocarbon dates should be expected, given the dramatic effects of the proposed impact: “The asteroid impact… …would alter the environment catastrophically through a hierarchy of interlinked events and processes, many of which could lead to an increase in the distribution of radiocarbon dates relating to the event. Ancient forests might be felled, tsunamis, earthquakes and landslides might mix and redeposit soils, and old sources of carbon might be redistributed. Even if some of these catastrophic processes might be modelled, there will always remain some doubt about the suitability and completeness of such models.”
HEA: Jorgeson et al. (2020) considered such a catastrophic event, the Laacher See volcanic eruption. The eruption felled trees, created a temporary lake through damming of the Rhine Valley, produced a 50-m thick tephra near the eruption center, and generated 1-m thick pumice deposits up to 120 km from the volcano (Baales et al., 2002; Bogaard and Schmincke, 1985). These processes left unambiguous features visible on Central Europe’s modern landscape. Even with these dramatic eruption effects, the Laacher See tephra contains radiocarbon samples consistent with simulations of a synchronous event (Jorgeson et al., 2020). By contrast, evidence for the catastrophic geomorphic processes suggested by Sweatman are lacking for the proposed YDB impact (Sections 3.3, 13.7). Impact proponents, in essence, argue that the impact produced global catastrophic effects far exceeding those of the Laacher See eruption, while paradoxically leaving no evidence for changes to the landscape. To our knowledge, YDIH proponents have not offered evidence for impact related tsunamis or earthquakes.
This criticism is a strawman argument. HEA invent evidence that they suggest must be present and then claim its absence refutes the YDIH. In fact, it is not yet clear what kinds of geomorphic processes accompanied the YD impact. For example, airbursts can be expected to lead to significant redistribution of sediments in a local area, which in turn could lead to significant variability in radiocarbon dates of the YDB, without leaving an obvious crater.
In any case, as already stated, it is simply impossible to know with any confidence whether the discrepancy between Jorgeson et al.’s (2020) models and the YDB radiocarbon data are caused by asynchroneity or model inadequacies, and this uncertainty can never be removed. Regardless of how sophisticated their models become, there will always be some doubt of unknowable likelihood that their models have not adequately accounted for all the processes involved. Therefore, Jorgesen et al.’s (2020) research program is not sensible.
HEA: In the third objection, Sweatman (2022) questions the very idea of modeling the YDB radiocarbon dataset. Since every physical process relating to chronology cannot be known with certainty, he argues that any unexplained variation in radiocarbon dates is unproblematic. For example, regarding the modeling of old wood effects with an exponential distribution, Sweatman (2022, p 3) states that “the exact ‘old wood’ model for AC [Arlington Canyon] is unknown, nor is it known whether any exponential distribution with any value of λ is adequate” and Jorgeson et al. (2020) “did not explore all possible forms of ‘old wood’ model. They only discuss simple exponential forms.”
HEA: An “exact” model cannot be known for most physical processes as models are, by definition, reductionist representations of the physical world. There are infinite possible old wood models that could be defined; although there are theoretical reasons to expect the distribution of old wood effects to be approximately exponential (Nicholls and Jones, 2001).
Any “theoretical reason” to expect an exponential distribution of old wood effects will be based on several assumptions. None are provided by Jorgeson et al. (2020) or HEA, so the validity of the exponential old wood model used by Jorgeson et al. (2020) has not been justified. Moreover, as explained above, an old wood model with a decay rate of 100 years (the largest considered by Jorgeson et al. (2020)) is unlikely to be adequate for modeling old wood effects up to 450 years in a small sample. This is because the cumulative probability distribution for ages older than 450 years is then exp(-4.5) = 0.011, yet the sample size is ~ 10 data points. A 200-year decay constant would be more appropriate in this case since then the cumulative probability distribution for ages older than 450 years would then be 0.11 which is commensurate with the sample size. It is unclear why Jorgeson et al. (2020) limited their exponential decay model to a decay constant of only 100 years when it is clearly inadequate.
HEA: Consequently, the exponential distribution is a standard model for old wood effects as implemented in OxCal (Bronk Ramsey, 2009), the application used to estimate many chronologies in archaeology, paleontology, and paleoclimatology. Impact proponents themselves used OxCal to model the age of the hypothesized event, and the vast majority of their radiocarbon samples were modeled with exponential old wood offsets (Kennett et al., 2015a). While all models are imperfect and incomplete, the strength of the evidence produced by a model with well-justified assumptions can be probative.
As already stated, any disagreement between a model and real data that is assumed to be synchronous either reveals the assumption of synchroneity is invalid or the model is invalid. Without further evidence, neither can be known with any confidence and therefore Jorgeson et al.’s (2020) conclusions are unsupported. However, the statistical argument provided above shows that YDB sites are very likely synchronous. Thus, Jorgeson et al.’s (2020) models are very likely inadequate.
The key phrase above is “well-justified assumptions”. In fact, the assumptions underlying Jorgeson et al.’s (2020) model are not well-justified. Nor can they ever be, in principle, because however sophisticated their models become, the probability they are inadequate is unknowable. Nevertheless, we can easily identify some severe problems with their current set of assumptions. For example, the old wood decay constant they use is clearly too short. Moreover, it is clear that their entire methodology is flawed at the outset because single radiocarbon samples do not in principle provide a good estimate for the true age uncertainty in any sample. Instead, proper age-depth models at each site are preferred.
HEA: Jorgeson et al.’s (2020) simulations are not just broadly inconsistent with a synchronous YDB, they demonstrate that the likelihood of a synchronous event producing the dispersion seen in the YDB dataset is astronomically low.
This is false. They do not demonstrate asynchronicity since an alternative explanation for their results is that their model is inadequate, as discussed above. Indeed, given the presence of impact proxies in the boundary layer across four continents, it is almost certainly synchronous - see the statistical argument given earlier.
HEA: The simulations account for many sources of variability in radiocarbon dating; while there may be other sources that are not included in the simulation, they would likely have only marginal effects on the results.
HEA provide no evidence to support this statement, which cannot be justified. As the debate above shows, neither Jorgeson et al. (2022) nor HEA provide adequate justification for Jorgeson et al.’s (2020) models. Nor can they ever, in principle, do so.
HEA: Sweatman (2022, p 2) raises a final objection against Jorgeson et al. (2020) on the grounds that their simulation “does not explain the physical evidence for the YD impact event at numerous YDB sites found, and confirmed, on multiple continents as reported in dozens of papers.” Regardless of the numerous problems with the purported physical evidence and dating enumerated throughout this and other papers, the objective of Jorgeson et al. was not to “explain” the claimed evidence for a YDB impact, only to illustrate that the YDB radiocarbon record is statistically inconsistent with a synchronous event. Neither Sweatman nor other YDIH proponents have demonstrated otherwise.
The statistical argument provided above demonstrates that the YDB is almost certainly synchronous across four continents. Therefore, it is almost certain that Jorgeson et al.’s (2020) modeling is flawed and several reasons for this are provided above. In any case, their research program is not sensible.
6.7. Summary of HEA’s Sections 5.3-5.8
Meltzer et al. (2014) fail to record uncertainty estimates for the coefficients of their linearly regressed age-depth models which in any case erroneously use calibrated radiocarbon data. Therefore, no conclusions can be drawn from their work, and it should be retracted. HEA consider these coefficients an unnecessary technical detail. On the contrary, they are crucial. This is demonstrated here specifically for Abu Hureyra but the same problems affect all their analysis.
This view is further amplified by HEA’s repeatedly unsound statements concerning uncertainty in radiocarbon measurements. These include criticism of the Bull Creek nanodiamond data of Bement et al. (2014) and Kennet et al. (2009). However, these data sets are clearly consistent (except possibly for a unit typo). HEA also debate the potential synchroneity of the Ussello/Finow charcoal-rich layer. But HEA provide no evidence that this charcoal horizon is not synchronous across many sites in Western Europe.
Likewise, Jorgeson et al. (2020, 2022) fail to recognize the limitations of their own models which were clearly pointed out by Sweatman (2021, 2022). Indeed, their research program is not even sensible. HEA also fail to recognize these limitations.
Altogether, this section of HEA is a Gish gallop, i.e. a long series of weak or false arguments. The reasons for this are obvious; their understanding of the logic required to properly test the YDIH and their analysis of uncertainty in experimental measurements are significantly lacking.
7. Rebuttal of other Sections of HEA
HEA frequently distort the facts to make their case. In addition to those already presented in earlier sections, here we detail several examples of their distortions and provide line-by-line comments in italics.
7.1. The updated YD impact scenario
In “Section 7: Multifarious YDB Impact Scenarios” of HEA we read;
HEA: As noted by Boslough et al. (2012, p 13) “there is not one single Younger Dryas (YD) impact hypothesis but several that conflict with one another regarding many significant details.” This is due to the fact that different impact scenarios are necessarily required by YDIH proponents to explain the disjointed contradictory evidence that is purported to support an Impact.
The above quote is from Boslough et al. (2012). There have been major developments in the YDIH since its inception including an updated impact scenario described by Wolbach et al. (2018a); that of a previously disrupted comet. HEA must ignore this work to make their statement above, despite knowing it very well.
Such updates are a normal feature of science. It is natural to adjust or fine tune a hypothesis as evidence accumulates, particularly an abductive hypothesis such as the YDIH. For example, see Figure 11 above. Boslough et al.’s (2012) statement is far out of date.
HEA: Firestone and Topping (2001, p 15) speculated a supernova shock wave “gouged out” the Carolina Bays to explain purported abnormal ratios of uranium isotopes and elevated plutonium at Clovis sites.
This is not the YDIH, which was first published in a peer-reviewed scientific journal by Firestone et al. in 2007, several years later. It is important that we address the impact hypothesis itself, not earlier speculations. The YDIH depends in no way on the origin of the Carolina Bays, which are something of a scientific mystery. HEA’s observation is a ‘strawman’ fallacy because it criticizes something which is not the YDIH as if it is.
Later in the same section we read…
HEA: However, most YDIH proponents report microspherules have terrestrial elemental composition (see Section 10), inconsistent with an airburst/impact not penetrating the ice sheet.
The updated impact scenario in Wolbach et al. (2018a, b) involving a previously fragmented comet specifically allows for a wide range of impact sites on multiple continents. They are not confined to the Laurentide ice sheet. Clearly, the microspherules were likely generated by these impacts. Once again, to make their statement above HEA must ignore this updated scenario despite knowing it very well.
Clearly, the microspherules were generated either by a ground impact or an airburst with sufficiently low altitude to melt and/or vaporize the ground. Such impacts are expected to generate microspherules with mainly a terrestrial composition, but some will have a meteoritic component, exactly as observed.
HEA: Proponents of the YDIH claim that purported Pt anomalies at the YDB (Section 11) are strong evidence of meteoritic material and an ET event (e.g., Moore et al., 2017; Sweatman 2021; Powell 2020, 2022) and ardently cite measurements of Greenland ice by Petaev et al. (2013a).
The word “ardently” is unnecessary, unscientific, and a pejorative. It should not be included in a scientific paper.
HEA: However, that Pt anomaly would require a surface impact not an airburst,…
This would be an interesting observation, if true, since it would imply that a YDB crater should exist. However, no arguments or evidence are cited to support this assertion. We expect instead that airbursts and meteoric ablation are capable of producing the observed long-lived atmospheric platinum anomaly since they can both reach temperatures sufficient to vaporize cometary material.
Indeed, during an airburst, the bulk of the meteoritic component is dispersed into the atmosphere (Schultz et al. 2022). The remaining meteoritic components are incorporated into impact minerals when introduced to the surface by the hot airburst plume. This process is different to a crater-forming impact, where meteoritic components are incorporated into the impact melt and shock products directly and thus dispersal via the atmosphere is less efficient. Therefore, we reject HEA’s claim that a surface impact is required to produce the observed platinum anomaly.
HEA: … and the lack of an identified YDB crater is again a serious problem for the YDIH.
The lack of a crater thus far is not yet a problem because the evidence can be explained by airbursts alone, although some would need to be very low altitude. However, a crater-forming ground impact remains a distinct possibility. Note that similar observations of microspherules, nanodiamonds and PGE abundances were made over ten years in advance of the discovery of the Chicxulub crater. Moreover, even young craters can be elusive if they are on the seabed, which is most likely given that oceans cover most of Earth’s surface. Earlier research by Osinski et al. (2008) has shown that low-altitude airbursts can take place without the formation of craters.
7.2. Coherent catastrophism
HEA: In attempt to explain skulls buried with microspherules, Hagstrum et al. (2017) with Firestone as a coauthor propose yet a different impact scenario where impacts/airbursts repeatedly occurred from ~46 kyr to ~11 kyr BP causing the megafauna extinctions over that time (Section 3.2). This contrasts with most YDIH versions that speculate a single impact event involving multiple fragments occurred at the YD/GS-1 onset and caused the megafauna extinctions.
Here, HEA demonstrate that they know that the favored impact model is that of a previously disrupted comet.
Moreover, the impacts suggested by Hagstrum et al. (2017) are obviously not directly related to the YDIH. They are clearly offering a different impact hypothesis where the only potential connection to the YDIH is through coherent catastrophism. That is, if the YD impact occurred, then a reasonable hypothesis is that it was a consequence of coherent catastrophism, which in turn suggests other large impact events over the last 20-30 thousand years or so are much more likely than recognized.
Later in the same section we read;
HEA: Sweatman (2021) castigates “Holliday et al. (2014), with Boslough as co-author” for ignoring “coherent catastrophism” (p 18). Holliday et al. (2014) ignore it because “coherent catastrophism” is a speculative hypothesis that is unsupported by observational data and inconsistent with the cratering record.
HEA provide no evidence to support their assertion that coherent catastrophism is a “speculative hypothesis that is unsupported by observational data and inconsistent with the cratering record”. In fact, observational data shows strong support for the notion of coherent catastrophism (for example, see Ferrin & Orofino (2021) and other sources below). Furthermore, the impact rate derived from Earth’s cratering record and other sources, like space surveys and lunar cratering, is an average over many hundreds of millions to billions of years. Yet the true impact rate is expected to fluctuate significantly on various timescales and is not expected to be constant. This is because giant comets in Earth-crossing orbits will occasionally increase the impact rate. This is coherent catastrophism.
To be clear, coherent catastrophism applies generally to all situations where a comet decays in an Earth-crossing orbit. Since we know that giant comets exist in the outer solar system among the population of centaurs and we know they have unstable orbits because of the presence of the outer planets, it is inevitable that they will occasionally adopt Earth-crossing orbits (see, for example, Horner et al. (2004), Napier et al. (2015), Galiazzo et al. (2019)). Simulations reveal we can expect a centaur to form an Earth-crossing orbit every ~ 1000 years assuming the centaur population is replenished by objects from outside the planetary orbits. Most of these Earth-crossers will be small and not lead to significant changes in Earth’s impact risk. However, a giant centaur will occasionally significantly perturb Earth’s bombardment risk. Thus, coherent catastrophism is inevitable, and not a speculative theory.
Evidence that this has occurred recently, within the last 20-30 thousand years or so, is provided by the existence of the massive Taurid meteor stream and the relatively high density of the zodiacal dust cloud.
Napier et al. (2013) already responded to Boslough (2013) on this subject;
“Boslough et al. (1) offer no alternate explanation for ~10 million tonnes of Younger Dryas spherules recovered from 18 sites across ~50 million square kilometers of North America, Europe, and the Middle East (2). In addition, the authors claim that our hypothesis “demonstrates a misunderstanding of comets.” However, the misunderstanding is theirs alone, because the model they criticize is their own creation and not the one we adopt, which derives from a substantial body of comet literature (e.g., ref. 3).
Most Earth-crossing comets arrive from the Oort cloud and the transneptunian region by way of the centaur population, an unstable reservoir orbiting between the giant planets that feeds the Jupiter family and Earth-crossing populations. From the size distribution of centaurs, as revealed by the Near-Earth Object Wide-field Infrared Survey Explorer space telescope (4), we find that there should be 30 centaurs >100 km in diameter with an average semimajor axis of ≤18 astronomical units (au) at any time. About half of them become Jupiter-crossing within 500,000 y, and 1 in 10 enter Earth-crossing, short-period orbits in the ecliptic plane, usually repeatedly (5). About one such entry occurs per 25,000 y, with a mean duration 2,000 y, although comets that reach sub-Jovian orbits achieve relative stability and much longer dynamical lifetimes. It is, therefore, not surprising to find the fossil remains of a large, recent, short-period comet in the near-Earth environment. There is such a system, comprised of about 15 correlated meteor streams containing some of the larger near-Earth “asteroids” (~2–5 km wide), as well as comet Encke at 5 km in diameter. The whole system is embedded in a broad swath of meteoroidal material dominating the zodiacal cloud. The mass and dispersion of this material indicates that the progenitor comet was initially 100 km across and began to break up at least 20,000 y ago (6). This system’s mass is far larger than the entire current near-Earth asteroid system.
Most meteor streams originate from discrete fragmentation events rather than gradual sublimation, and the substreams of the complex show that the progenitor comet also followed this route. Breakups tend to happen just after perihelion passage, yielding clusters of fragments with, at 1 au, about 10,000 times the cross-sectional area of the Earth. Hundreds of such clusters (~10^18 g) may be temporarily created over the lifetime of the comet. Passages through one or two are reasonably probable events, and are capable of yielding Younger Dryas boundary-like phenomena (3); no “exquisite timing” is required, as claimed by Boslough et al. (1).”
HEA: Sweatman (2021) misunderstands or misrepresents the objections by orbital dynamics and impact physicists to the extreme version of the coherent catastrophism hypothesis, which postulates without evidence that the current impact rate is grossly underestimated.
As explained above, the evidence for coherent catastrophism is overwhelming. The “objections by orbital dynamics and impact physicists” are not described or cited by HEA. Nor is the “extreme version” of coherent catastrophism described. In fact, cometary scientists who work in this area use the giant comet model, i.e. coherent catastrophism, as their working model for the evolution of the Taurid meteor stream (see sources below).
HEA: As with many of the YDIH proponents’ exaggerated claims, there is a grain of truth to dynamic arguments for resonant Taurid swarm and the possibility of transient increases in airburst rates when it intersects with Earth. Far from ignoring coherent catastrophism, Boslough and Brown (2018) spearheaded an effort for an observational campaign in the summer of 2019 to conduct astronomical surveys with the aim of detecting possible objects in the hypothetical Taurid resonant swarm, which is foundational to coherent catastrophism. They used computational models to show that the Tunguska airburst effects were indeed consistent with the trajectory of a Beta Taurid.
If HEA do, in fact, accept the basic idea of coherent catastrophism and have even published in this area, then their position on coherent catastrophism above where they describe it as a “speculative hypothesis that is unsupported by observational data and inconsistent with the cratering record” appears to be inconsistent and is hard to explain. One possibility is that HEA seek to associate an unspecified ‘extreme version’ of coherent catastrophism with the YDIH while simultaneously supporting the basic premise of coherent catastrophism. Without further elaboration, HEA’s argument seems to simply be a rhetorical tactic.
HEA: Clark et al. (2019) subsequently calculated the observability of the postulated resonant swarm and recommended an observational campaign to document it in the summer of 2019. There were no reports of significant discoveries of predicted Taurid swarm objects in 2019, however. The lack of observational evidence for the predicted high-density swarm of such objects is inconsistent with the models of Napier (2010, 2019) that were invoked by Sweatman (2021) in support of the YDIH. Coherent catastrophism is also discussed in Section 5.2.
Since Boslough “spearheaded” calls for this observational campaign and is also a co-author of HEA, they must surely know the 2019 campaign was not completed and no papers have been published about it. Thus, HEA’s implied suggestion that it did take place seems to be incorrect. However, the 2015 observational campaign, not mentioned by HEA, had already confirmed the presence of the predicted Taurid resonant swarm whose existence is generally accepted among cometary scientists (see below).
Moreover, HEA seem unaware of developments since the non-event of the 2019 observations. Indeed, Boslough’s co-author in 2018, P.G. Brown, co-authored a couple of papers in 2022, i.e. 6 months before HEA submitted their work. In one paper, Egal et al. (2022a, b) conclude in their abstract;
“Our analysis thus suggest that with this specific dynamical history, 2P/Encke is the sole parent of the four major TMC showers that have ages from 7 to 21 ka. Our modelling also predicts that the 2022 Taurid Resonant Swarm return will be comparable in strength to the 1998, 2005, and 2015 returns. While purely dynamical models of Encke’s orbit –limited by chaos –may fail to reveal the comet’s origin, its meteor showers may provide the trail of breadcrumbs needed to backtrack our way out of the labyrinth.”
In another paper, Egal et al. (2022a, b) conclude in their abstract;
“We provide an overview of the observational properties of the four major Taurid showers, namely the Northern and Southern Taurids (#017 NTA and #002 STA), the βTaurids (#173 BTA), and the ζPerseids (#172 ZPE). … Optical measurements have previously recorded enhanced STA activity and increased fireball rates caused by the return of a swarm of meteoroids trapped in the 7:2 mean motion resonance with Jupiter. However, we find no presence of the swarm in radar data, suggesting that small meteoroids are removed from the resonance faster than fireball-producing meteoroids.”
Moreover, Ferrin and Orofino (2021) conclude in their abstract;
“Using the Secular Light Curve (SLC) formalism (Ferrín, 2010), we have catalogued 88 probable members of the Taurid Complex (TC). 51 of them have useful SLCs and 34 of these (67%) exhibit cometary activity. This high percentage of active asteroids gives support to the hypothesis of a catastrophe that took place during the Upper Paleolithic (Clube and Napier, 1984), when a large short-period comet, arriving in the inner Solar System from the Kuiper Belt, experienced, starting from 20 thousand years ago, a series of fragmentations that produced the present 2P/Encke comet, together with a large number of other members of the TC. … Other components of the complex are groups of meteoroids, that often give rise to meteor showers when they enter the terrestrial atmosphere, and very probably also the small asteroid that in 1908 exploded in the terrestrial atmosphere over Tunguska. What we see today of the TC are the remnants of a very varied and numerous complex of objects, characterized by an intense past of collisions with the Earth which may continue to represent a danger for our planet.”
Meanwhile, Devillepoix et al. (2021) conclude in their abstract;
“The Desert Fireball Network observed a significant outburst of fireballs belonging to the Southern Taurid Complex of meteor showers between 2015 October 27 and November 17. At the same time, the Cameras for Allsky Meteor Surveillance project detected a distinct population of smaller meteors belonging to the irregular IAU shower #628, the s-Taurids. … We confirm that the meteoroids have orbital periods near the 7:2 mean motion resonance with Jupiter. The mass distribution of this population is dominated by larger meteoroids, unlike that for the regular Southern Taurid shower. … This supports a model for the Taurid Complex showers that involves an ongoing fragmentation cascade of comet 2P/Encke siblings following a breakup some 20,000 yr ago.”
In addition, Beech et al. (2020) state that;
“… the Taurid Complex is generally taken to be composed of the debris derived from the break-up of a large 50-km sized comet (possibly Kuiper belt object) some 20-30,000 years before present [38-40]. … Furthermore, a component of the Taurid meteoroid stream is known to be in a 7:2 mean motion resonance with Jupiter, and this not only restricts the dispersal of the meteoroids within the stream, but it also controls the appearance of distinctive fireball outburst events [42, 44, 45] – these being times at which an enhanced flux of particularly large meteoroids encounter the Earth. The beta- Taurid stream is particularly interesting with respect to the topic under discussion here since it has been identified as the parent stream to the Tunguska impactor [38, 46, 47].”
This recent body of research clearly shows that coherent catastrophism, which describes the possibility that giant comets can decay in Earth-crossing orbits, is the generally accepted model that describes the appearance and evolution of the Taurid meteor stream and therefore implies a significantly increased risk of impact to Earth over the last 20-30 thousand years or so. But HEA label it instead a “preposterous fringe idea” in Section 13.2 “More Pseudoscience (Fringe) Evidence and Conjecture”. Their statement is itself preposterous.
Later in section 7 we read…
HEA: The lead author of that paper who is also a coauthor of this review (MP) now attributes it to a small local event, probably the one associated with the Cape York meteorites as suggested by Boslough (2013).
HEA already claimed (see above) that a crater-forming ground impact is required to generate the long-lived atmospheric platinum anomaly. Since the Cape York meteorite is not associated with any crater, according to their own position it could not have produced the platinum anomaly. In fact, the Cape York strewn field is well characterized, with as many as 9 fragments weighing up to 31,000 kg recovered, none associated with an impact crater or plunge pit. Analysis by Beech et al. (2020) specifically concerning a potential relation between the Cape York Meteorites, the Hiawatha & Paterson Craters, and the YDIH, found that all three likely had no relationship. Finally, a small local event over Greenland should not be expected to produce a strong platinum anomaly more than 10,000 km away in Patagonia (Pino et al. 2019) or more than 14,000 km away in South Africa (Thackeray et al. 2019).
7.3. Microspherules
HEA: Even though small (mm to cm-size), often glassy impact melt bodies (“spherules”) may be ejected from the cratering site during impact, and form (often geographically extended) ejecta deposits, their existence as melt particles is not as such diagnostic for impact, and their identification as impact products depend on association with other, confirmed impact-produced features, such as shock effects.
YDIH proponents use a diagnostic process based on many characteristics, including surface textures and geochemistry using SEM and XRD, to reach their conclusions that most of these microspherules are very likely impact generated. They do not simply rely on them being small, round and glassy. Indeed, morphological analysis of the fine surface textures of YDB microspherules is used in conjunction with geochemical composition to determine that they can only have formed at very high temperatures during a ground impact, airburst, or nuclear detonation. During these events, terrestrial material is melted and vaporized before becoming airborne and condensing/quenching rapidly, producing aerodynamic forms including spheres and teardrops, traditionally referred to as tektites or microtektites. Differential condensation rates of elements in the plume can result in silicate spheres with thin crusts of iron condensate, which are found both in the YDB and following nuclear detonations (Genda et al. 2021). Moreover, the general view is that most of the impacts were airbursts, perhaps quite low in altitude, and not often (or perhaps at all) crater-forming. Thus, the requirement that only evidence for crater-forming impacts is acceptable is not appropriate. Consider that, if a YD airburst actually did occur, according to HEA’s criteria it would be impossible to scientifically verify this. Thus, their approach can be rejected; it is not scientific.
HEA: Tektites and microtektites are the best-known and most-studied of these ejecta deposits (e.g., Koeberl, 1994), but a variety of other glass-rich ejecta deposits, have also been noted. The identification of such glasses as impact or non-impact products is difficult and commonly controversial as discussed in the extensive review by French and Koeberl (2010).
French and Koeberl (2010) is focused on ground impacts, not airbursts. Note that the title of their work is “The convincing identification of terrestrial meteorite impact structures…”. Also note that the word “airburst” is mentioned only twice in their paper, both times in the context of the YDIH. The word “crater”, however, is mentioned 272 times. Clearly, their work is not appropriate for critiquing the YDIH, except where ground impacts may have occurred.
Especially, French and Koeberl (2010) do not critique the detailed, simultaneous, diagnostic features of microspherules described by YDIH proponents. Simply saying their identification is “difficult” is insufficient.
However, several quotes from French and Koeberl (2010) are worth highlighting. For example; “… siderophile-element anomalies may be absent in spherule layers” and
“… the use of microspherules alone as impact indicators requires careful and meticulous work to demonstrate conclusively that they are: (1) natural and (2) impact-produced” and “Like other impact melts, droplet spherules generally preserve no evidence of shock processes or of their original ultrahigh-temperature origin. There are rare exceptions: inclusions of lechatelierite, coesite, and shocked zircon, which establish an impact origin directly…”
Clearly, French and Koeberl (2010) accept that PGEs may be absent in impact microspherules and that it is possible to diagnose an impact from microspherule evidence alone given suitable criteria. Regarding the latter point, French and Koeberl (2010) state;
“Petrographic characteristics of impact-produced spherules include (Simonson, 2003, pp. 52–62):
a restricted size range (typically 60–2000 μm);
the presence of abundant splash-form shapes (spheroids, dumbbells, teardrops, etc.) indicative of melting;
crystallization textures that develop inward from the rims rather than outward from a central core;
an absence of associated typical nonspheroidal fine volcanic materials (e.g., glassy shards, glassy filaments [“Pele’s Hair”], corroded phenocrysts, or volcanic rock fragments); and
the presence of associated definite shock effects, such as particles containing lechatelierite or grains of shocked quartz with PDFs (but see also Marini and Rauka, 2004).
Geochemical indicators of impact-produced spherules include (Montanari and Koeberl, 2000, pp. 71–77, 118–123; Simonson, 2003; Simonson and Glass, 2004):
original compositions corresponding to natural crustal target rocks or to mixtures of such rocks;
compositions unlike typical volcanic rocks;
an absence of exotic compositions, e.g., rare-metal alloys, hydrocarbons or enrichments in non-meteoritic elements such as Ba, Ti, Mn, Pb, etc., which, if present, suggest a natural or artificial terrestrial origin; and
the presence of anomalous concentrations of projectile-related chemical elements (e.g., Ir and the other PGEs) or isotopic signatures (e.g., Os, Cr).”
The above criteria mirror those used in Bunch et al. (2012), Wittke et al. (2013) and Moore et al. (2020). Lechatelierite, and elevated levels of platinum and iridium are found in YDB objects at Abu Hureyra, confirming a ground impact or airburst there. Elevated platinum or lechatelierite are noted at ~ 30 other YDB sites, strongly suggesting impacts at those locations too. The similarity of microspherules across many more YDB sites strongly suggests they were subjected to ground or airburst impacts too. The relative lack of craters or shock metamorphism discovered at YDB sites to date points mainly to airbursts, but this could change.
HEA: Some studies, in which such spherule layers have been carefully examined by geological, petrographic, and geochemical techniques, provide strong evidence that they formed by meteorite impact events. In most cases, however, the confirmation of the impact origin did not come from the spherules themselves, but from associated minerals or geochemical anomalies.
This appears to be very similar to a section in French and Koeberl (2010), which reads;
“Recent studies, in which such spherule layers have been carefully examined by geological, petrographic, and geochemical techniques, have provided strong evidence that they have been formed by meteorite impact events (Margolis et al., 1991; Montanari and Koeberl, 2000, Chs. 2,3; Simonson, 2003; Simonson and Glass, 2004). However, in most cases the confirmation of the impact origin did not come from the spherules themselves, but from associated minerals or geochemical anomalies.”
HEA: “Microspherules are not, by themselves, diagnostic indicators of impact events, because similar objects can be produced by a wide range of geological and artificial processes… Identification of microspherule-bearing layers as impact ejecta needs additional evidence: geological context, association with genuine quartz PDFs [planar deformation features], high-pressure minerals, or definitely extraterrestrial siderophile-element anomalies” (French and Koeberl, 2010, p 151-152).
This appears to contradict the direct quote above from French and Koeberl (2010) which states that impacts can be diagnosed from microspherule evidence alone. Moreover, YDIH proponents have presented detailed diagnostic evidence for impact microspherules that mirrors that suggested by French and Koeberl (2010). To counter their claims, HEA must provide equally detailed counter-evidence. They need to be specific about what other kind of process can create the microspherules found at many YDB sites with the same set of characteristics. If there are none, they should conclude that only an ET impact is known to produce microspherules with all these characteristics.
Later in Section 10 of HEA we read…
HEA: As summarized by French and Koeberl (2010, p 145-147), “There are several major problems in attempting to use spherules as independent evidence for meteorite impact events… Spherules alone do not provide diagnostic evidence of origin by impact…
Again, this contradicts other statements in the same paper - see above. ET microspherules are accepted by themselves as evidence of a cosmic event by French and Koeberl (2010).
HEA: … Like other impact melts, droplet spherules generally preserve no evidence of shock processes or of their original ultrahigh-temperature origin….”
It is especially notable that HEA terminate this quote from French and Koeberl (2010) at this point, because the next line in French and Koeberl (2010) reads; “There are rare exceptions: inclusions of lechatelierite, coesite, and shocked zircon, which establish an impact origin directly…” It is not clear exactly why HEA terminate their quote at this point, but clearly had they continued they would have contradicted themselves.
As already noted, lechatelierite has been found in the YDB at Abu Hureyra and at several other YDB sites on other continents.
7.4. Daulton et al.’s (2010) nanodiamond samples
In “Section 4.1: Arlington Canyon Confusion” of HEA we read;
HEA: Wittke et al. (2013a, supplemental materials) incorrectly assert that Scott et al. (2010; 2017) and Daulton et al. (2010; 2017a,b) sampled the wrong localities at Arlington Canyon.
How can Wittke et al. in 2013 incorrectly assert that Scott et al. in 2017 and Daulton et al. in 2017 sampled the wrong localities? After all, they are not time travelers! Clearly, they were referring to the samples used in Daulton et al. (2010) whose location is specified in Scott et al. (2010).
HEA: This flawed assertion was echoed by Kinzie et al. (2014), Sweatman (2021), and Powell (2020, 2022).
HEA: Kinzie et al. (2014, p 477) wrote, “Their incorrect stratigraphic locations apply to all those investigations, explaining their inability to detect YDB NDs [nanodiamonds], cosmic- impact spherules, and ND-rich carbon spherules at Arlington Canyon.” In Sweatman (2021) much of the ‘discussion’ of the non-reproducibility of the nanodiamonds focuses on this incorrectly perceived misidentification of sampling localities rather than addressing any of the substantive criticism (similar to the approach taken in dismissing the many critical problems of dating documented by Meltzer et al., 2014; Section 5.3).
As we saw in the previous section, Meltzer et al. (2014) is fundamentally flawed. Moreover, neither Meltzer et al. (2014) nor HEA have shown that any of the “many critical problems of dating documented by Meltzer et al., 2014; Section 5.3)” are significant since they failed to produce age-depth models which include the uncertainty in the coefficients of their linear fits to the radiocarbon data.
HEA: Impact proponents make much of the spatial coordinate problem that was in fact due to a failure by Kennett et al. (2009b) to state the associated Datum. For example, Sweatman (2021, p 9) not only reproduces criticisms that had already been addressed but failed to understand what was said.
Wittke et al., [2013a] and Kinzie et al. (2014) show… that Daulton et al. (2010) did not, in fact, sample the same site as Kennett et al. (2009b) at Arlington Canyon – instead their samples with labels SRI-09 were obtained from several different locations separated by up to 7000 m from the site sampled by Kennett et al. (2009b). Scott et al. (2017), with Daulton as co-author, later refuse to admit this error, pointing to photographs that show that they did indeed sample the same sediment bank as Kennett et al. (2009b).”
Just so that it is clear what is being debated here, the original samples are described in Daulton et al. (2010);
“Carbon spherules were isolated from the same locality (Arlington Canyon, Santa Rosa Island, CA) reported to contain hexagonal nanodiamonds (16). Kennett et al. (14, 16) dated the entire basal 5 m of the Arlington YD sequence within the 1σ range 13,100–12,830 cal yr B.P. and reported nanodiamonds in the deepest meter thick layer (16). In contrast, we obtained calibrated radiocarbon dates spanning >5;000 years over that same 5-m sequence. From that sequence, we examined two specimens for nanodiamonds (from the lowest meter) dating to 12,766–13,044 and 13,379–13,560 cal yr B.P. (1σ range).”
From the table in Figure 12 the only Arlington Canyon samples examined for nanodiamonds by Daulton et al. (2010) are those labeled SRI-09028 and SRI-09-29c. The precise location of those samples is given in the supplementary information of Scott et al. (2010).
Wittke et al. (2013) provided a map of the location of all the AC samples in Daulton et al. (2010) and Scott et al. (2010) (shown in Figure 13 below). Note this map uses the specified coordinates and projection for each sample. The ones examined for nanodiamonds in Daulton et al. (2010) correspond to points C and D in Figure 10. Clearly, they are not the same location as the Kennett site (AC003). Nevertheless, HEA insist that they are.
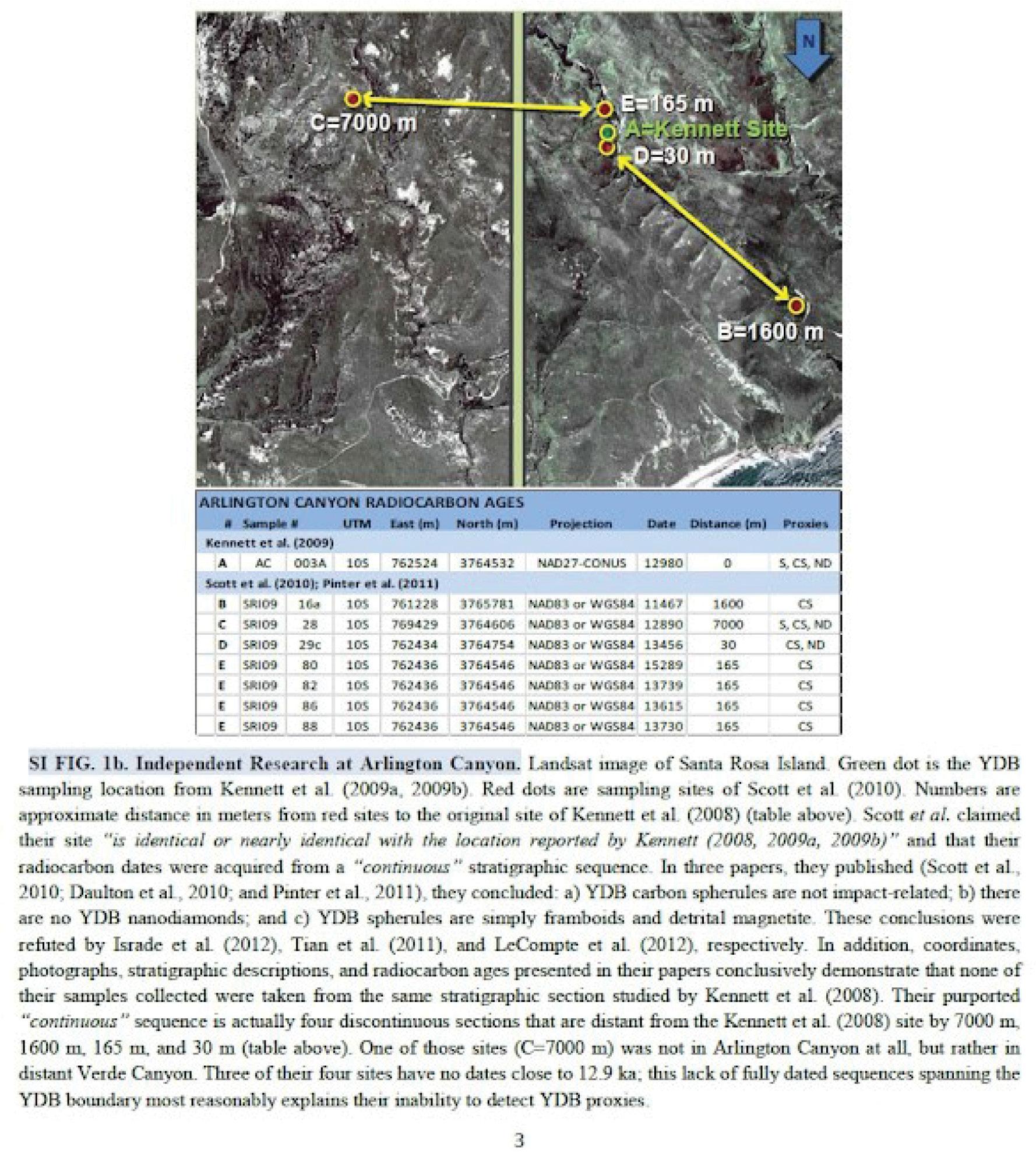
Extract from the appendix of Wittke et al. (2013) showing the location of the samples used by Daulton et al. (2010).
HEA: Scott et al. (2017, p 44-45) clearly explained the situation, which we further clarify for emphasis. “Arlington Canyon has featured centrally in results suggesting a global-scale impact drove broad changes at the onset of the Younger Dryas (the YDIH). Wittke et al., [2013a] assert that we did not study the same section as theirs (AC-003).” This is not true.
This lacks precision. Specifically, Wittke et al. (2013), Sweatman (2021) and others assert that Daulton et al. (2010) did not examine the same section as Kennett et al (2009), i.e. (AC-003), for nanodiamonds. HEA consistently muddy the water by conflating Daulton et al. (2010) with much later papers.
HEA: While Kennett et al. ([2008a], 2009b) gave UTM coordinates without specifying the associated datum or map projection, we were able to navigate to [the general area which we searched and found] their published location using the North American Datum 1983 (NAD83) and found there the largest, best exposed, and most accessible outcrop in Arlington Canyon. Later we surmised that Kennett et al. ([2008a], 2009b) had used NAD27 (confirmed in Wittke et al., [2013a]). We subsequently measured, sampled, and dated the small section at that location.”
This is vague. Which samples are HEA referring to here? We are only interested in the location of samples SRI-09 that were analyzed by Daulton et al. (2010) for nanodiamonds.
HEA: Further, Scott et al. (2017, p 37) explained: “Wittke et al., [2013a] claim that ‘coordinates, photographs, stratigraphic descriptions, and radiocarbon ages presented in their papers… conclusively demonstrate that none of their samples collected were taken from the same stratigraphic section studied by Kennett et al., [2008a].’ On the contrary, our Locality III is identical to their locality AC003…
Once again, Wittke et al. (2013), Sweatman (2021) and others are clearly referring to the samples labeled SRI-09 analyzed by Daulton et al. (2010) for nanodiamonds. We can agree that Locality III is identical to locality AC-003, but no samples taken from Locality III were examined for nanodiamonds by Daulton et al. (2010).
Later in the same section we read.
HEA: Sweatman (2021, p 20) also asserts, “when attempting to reproduce purported evidence for a cosmic impact, it is important that similar samples from exactly the same stratum at the same site are taken. Daulton et al.’s (2010) search for nanodiamonds appears to be hamstrung by this issue, an error these researchers seem determined not to admit…” (emphasis added). Scott et al. (2017, figure S1) unambiguously establish that Scott et al. (2010; 2017) and Daulton et al. (2010; 2017a,b) sampled precisely the same section as field site AC003 of Kennett et al. (2009b).
Once again, Sweatman (2021), Wittke et al. (2013) and others were clearly specifically referring to Daulton et al. (2010). But HEA consistently conflate Daulton et al. (2010) with later papers, thus muddying the water. Nevertheless, this is HEA’s best evidence for showing that the location of Daulton et al.’s (2010) SRI-09 samples is the same as the Kennett site AC-003. They suggest this evidence is in Figure S1 of Scott et al. (2017). In fact, that figure is just a smaller part of Figure 2 from the main text of their paper, shown below in Figure 14 .
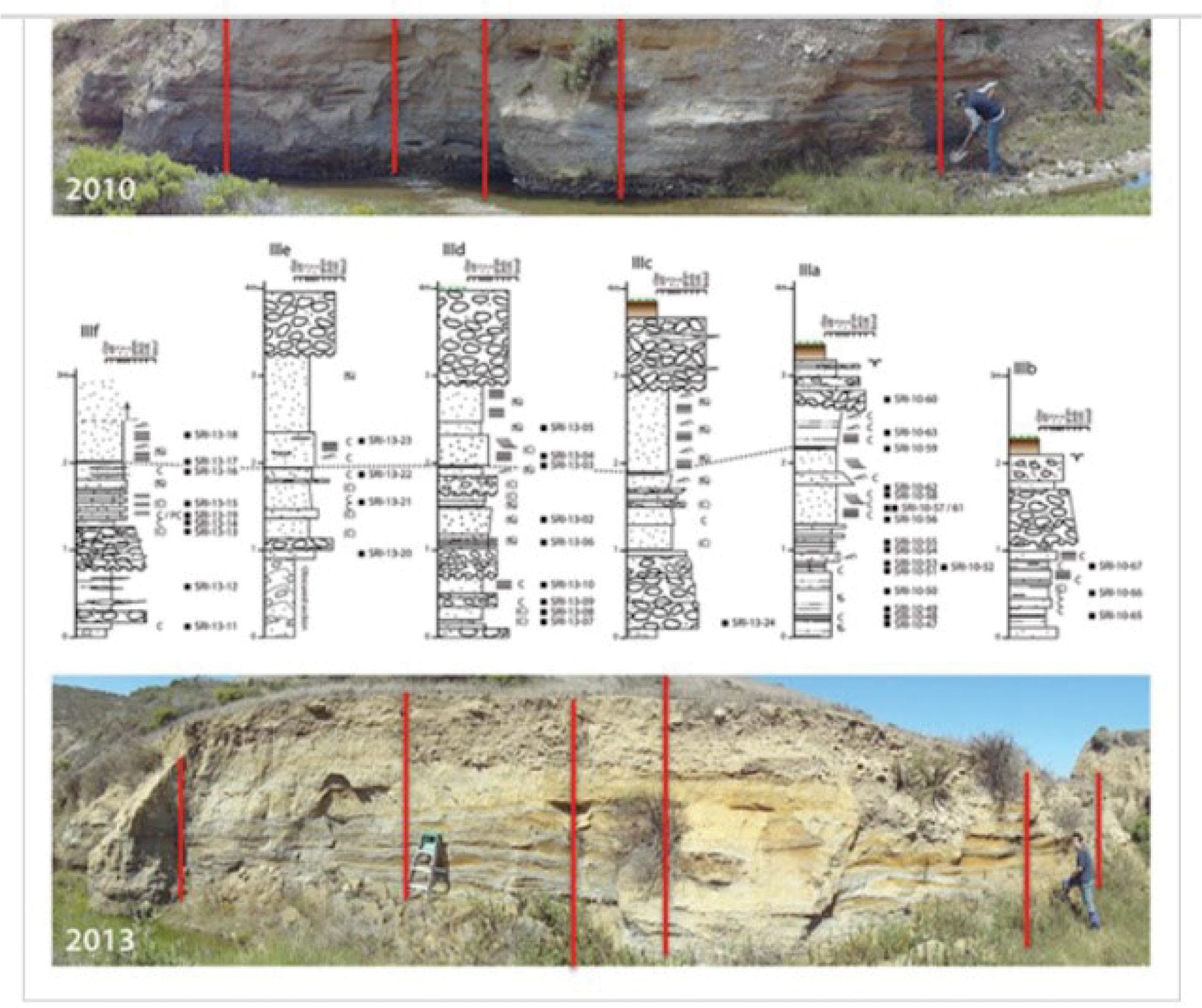
Figure S1 of Scott et al. (2017) showing the location of samples SRI-10 and later, but not samples SRI-09.
The photographs in Figure 14, corresponding to Figure S1 of Scott et al. (2017), do clearly show the same location as the Kennet site. However, these photographs do not list any of the samples labeled SRI-09 analyzed by Daulton et al. (2010) for nanodiamonds. Thus, HEA provide no evidence that the samples examined for nanodiamonds in Daulton et al. (2010) came from the same site as the Kennett site (AC-003). In fact, we know they didn’t, because their coordinates show they didn’t.
In Daulton et al. (2017) we read;
“Millimeter-scale carbonaceous spherules and/or their fragments were isolated from Arlington Canyon, Santa Rosa Island, California, sediments AC-003 (Kennett et al., 2008, 2009b) and SRI 09-28A from Locality III (Scott et al., 2010, 2016) that were dated to the YDB (12 800–13 100 and 12 718–13 079 cal a BP, respectively).”
First, note that Daulton et al. (2017) are claiming that sample SRI-09-28A is from locality III (i.e. AC-003), when the map coordinates given by Scott et al. (2010) show clearly that it is not. It is around 7 km from AC-003. Either Scott et al. (2010) is mistaken or this statement in Daulton et al. (2017) is wrong.
In summary, the above defense of Daulton et al. (2010) by HEA is misleading and confused. The samples labeled SRI-09 analyzed by Daulton et al. (2010) are frequently conflated with other samples analyzed in later papers, but these latter samples are not those of interest. Nevertheless, the map coordinates are very clear; either Daulton et al. (2010) failed to sample the same AC-003 site as Kennet et al. (2009) as indicated by the map and coordinates in Figure 13 above, or they did sample the correct site, but incorrect map coordinates were recorded by Scott et. al. (2010). If the latter is true, then Daulton et al. (2010) should explain why incorrect map coordinates were provided by Scott et al. (2010) and provide the correct coordinates. If they cannot do this, Daulton et al. (2010) should be retracted.
Later in the same section we read;
HEA: But even if it was not the “exactly the same stratum”, Sweatman’s (2021) assertion is ridiculous because impact proponents claim that at the YD/GS-1 onset a layer of impact markers was deposited across North America to Europe. If this were the case, then certainly an YDB-dated layer containing those markers would have covered the entire island of Santa Rosa.
YDIH proponents have never claimed the YD boundary layer is continuous across several continents, and no evidence is provided to support this claim. Probably, HEA’s misunderstanding here is caused by their own misinterpretation of comments made by various authors that the YDB stretches across or spans several continents etc., but no YDIH proponent has ever claimed the YDB is perfectly continuous. Of course, no such claim could ever be made, since the presence of the YDB will be influenced by its proximity to an ET impact site and a variety of local conditions.
In any case, regarding the samples analyzed for nanodiamonds by Daulton et al. (2010), sample SRI09-028 is charred wood (see the table in Figure 12 above) while sample SRI09-29A is dated to 13,341-13,619 BP (2-sigma), and therefore neither sample is expected to contain any nanodiamonds anyway, regardless of their location. Thus, not only did Daulton et al. (2010) sample the wrong locations, which they claimed were the correct ones, they also took the wrong samples. It is therefore no surprise that they did not find any nanodiamonds.
Daulton et al. (2010) was one of the earliest works that claimed evidence for the YDIH cannot be reproduced. Its conclusions have been influential and used many times by opponents of the YDIH to dispute the YDIH. Its flaws have been pointed out many times, but these are simply ignored by YDIH opponents.
7.5. Platinum
HEA: It is the extremely high Pt/Ir ratio at the Pt anomaly in the Greenland ice that “rules out mantle or chondritic sources of the Pt anomaly (Fig. 2). A further discrimination between Pt-rich crustal materials like Sudbury Footwall ore… and fractionated extraterrestrial sources such as Ir-poor iron meteorites… is difficult because of the comparable magnitude of the Pt/Ir fractionation in these materials. Circumstantial evidence hints at an extraterrestrial source of Pt, such as very high, superchondritic Pt/Al ratios at the Pt anomaly and its timing, which is clearly different from other major events recorded in the GISP2 ice core, including well-understood sulfate spikes caused by volcanic activity and the ammonium and nitrate spikes associated with biomass destruction” (Petaev et al., 2013a, p 12918).
Indeed, this all points towards an ET source, as expected. Note that Petaev et al. (2013) also state that;
“… both terrestrial and extraterrestrial high-Pt sources have substantially lower Pt/Ir ratios than those at the top of the Pt peak, implying either Pt-Ir fractionation during atmospheric processing of the Pt-rich materials or multiple injections of materials with different Pt/Ir ratios not sampled so far.”
HEA ignore this inconvenient statement, even though Petaev is a co-author.
In other words, the PGE composition detected is not a sure test for the origin of the ET matter because i) impact processes (and presumably many other environmental processes) can modify the composition detected, and ii) the impactor might be a highly unusual ET object. Nevertheless, the observed platinum abundance is consistent with an ET impact.
HEA: The later investigation of YDB sediments at Hall’s Cave and Friedkin sites by Sun et al. (2021, p 70) showed that highly siderophile element “analysis including Os isotope measurement is needed to provide a clear picture of the source of the geochemical signatures as either being extraterrestrial or mantle derived material.”
Sun et al. (2021) did not “show” this. It is simply the assumed basis for their investigation. As already stated, we cannot know the PGE composition of a specific comet in advance, although it is generally thought they are likely to be like primitive chondritic meteorites plus added volatile ices. Petaev et al. (2013) already indicated that impact processes can modify that apparent composition making identification of the source uncertain. That is, we have already seen that the GISP2 platinum signal is enigmatic. We can therefore expect the YDB PGE signal elsewhere to be enigmatic too. In particular, if atmospheric fractionation resulted in platinum enrichment relative to iridium in the GISP2 ice core, as suggested by Petaev et al. (2013), then it is also possible it resulted in enrichment of platinum relative to osmium. Therefore, analysis of osmium in sediment layers at YDB sites might not be very helpful since the signal might be very weak relative to platinum and quite unlike that expected for normal chondritic impactors. This agrees with the observation of Wu et al. (2013) who also failed to find a clear osmium signal at several YDB sites.
It is worth noting the normal boiling points of the six PGEs (in Celsius, from Wikipedia, boiling points of the elements): palladium: 2963; rhodium: 3695; platinum: 3825; ruthenium: 4150; iridium: 4130; osmium: 5012. If the impact fireball was sufficiently hot to vaporize a lot of the platinum, but insufficient to vaporize so much iridium and osmium, then we would expect to observe fractionation leading to depletion of iridium and especially osmium in atmospheric particles (aerosols) relative to platinum. This might then explain the relative PGE abundances seen by Petaev et al. (2013), Wu et al. (2013) and Sun et al. (2020).
Later in the same section we read;
HEA: For example, Os isotopes and PGE data of Sun et al. (2020; 2021) for sediments below, above and within the YDB layer from the Hall’s Cave and the Debra L. Friedkin site do show several Pt spikes, with one sample (BMC16_11.D – Sun et al., 2021) having very high Pt/Ir ratio of 1937 and very low Pt/Lu ratio of ~0.0007 due to the dominance of terrestrial silicate matter in sediments. Based on the Pt/Ir ratio alone, the nature of this Pt-rich and Ir-poor material cannot be resolved. It is the dominance of silicate matter in sediments that rules out usage of the Pt anomaly alone or even with Ir as a proxy of ET matter in them. Sweatman (2021, p 2) describes PGEs (“especially platinum itself”) as “the most robust impact proxies” but clearly this is not the case here unless a comprehensive analysis of PGEs and siderophile elements is performed. For example, a volcanic source of PGE anomalies at Hall’s Cave and the Friedkin site was deduced based on a wider examination of 187Os/188Os isotopic ratios as well as abundances of Os, Ir, Ru, Pt, Pd, and Re (Sun et al., 2020, 2021).
As already stated, osmium, if it is fractionated like iridium in the GISP2 ice core, might not be a useful guide to the origin of the platinum abundance. In fact, Sun et al.’s (2020) data suggests that there could be separate osmium-rich events (which they conclude are volcanic in origin) and platinum-rich events (which could be signaling an ET event) including a platinum-rich event at the YDB consistent with the YDIH.
Regarding the position of the YDB in Hall’s Cave, Stafford et al. (2009) state;
“The red clays from 151 to 153 cm and immediately preceding the lithologic contact contain an abundance of nanodiamonds (5 different allotropes), aciniform soot at 2400 ppm, magnetic spherules, and carbon spherules, all of which we interpret as evidence for a unique chronostratigraphic marker (YDB) in the Western Hemisphere. Because the age of this horizon is ~ 13,000 CAL BP, we interpret the age of the event as the beginning of the Younger Dryas cooling.”
The depths quoted for the YDB above refer to a single sediment column at one specific position in Hall’s Cave. But it is important to note that due to the undulating nature of the cave’s stratigraphy, the precise depth of the YDB at nearby positions in the cave might vary by a few cms.
Unfortunately, two measurements from Hall’s Cave taken by Sun et al. (2020) are missing in their report. Details of the complete set of measurements were provided to us by T.W. Stafford Jr. (2020), the principal investigator of the site and former coauthor of the study. Only 37 measurements are detailed in the Appendix of Sun et al. (2020) while 39 measurements were provided by Stafford (2020). The first missing sample, HC15_14 at 136 cm, is unremarkable. However, the 2nd missing sample, HC16_02 at 153 cm, has a quite remarkable platinum abundance of 1807 ng/g, the highest among all the measurements from Hall’s Cave. The osmium abundance for this sample is an unremarkable 25 ng/g, while the osmium 187/188 ratio is also unremarkable at 1.96 ± 0.1 (2-sigma). The sample at 153 cm is in contact with the expected position of the YDB at Halls Cave at 151-153 cm. Exclusion of this strong platinum-rich sample at 153 cm (HC16_02) requires some explanation by Sun et al. (2020). Its inclusion would have constituted evidence in support the YDIH. In fact, only by its exclusion are Sun et al. (2020)’s claims regarding the location of the YDB at Hall’s Cave and its relation to volcanism supportable.
It is also worth remarking on how the measurement campaigns were carried out by Sun et al. (2020). Their 2015 campaign yielded osmium-rich samples at 140 cm and 171-176 cm, but no PGE-rich samples near the YDB at 151 cm. The 2016 campaign yielded an additional platinum-rich sample at 143 cm corresponding closely to the end of the YD period, an additional osmium-rich sample at 155 cm, below the YDB, and the unreported platinum-rich sample at 153 cm at the base of the expected position of the YDB. Had Sun et al. (2020) stopped there, they would have confirmed a platinum abundance near the YDB without any osmium abundance. However, they completed an additional 2017 campaign focused at 151 cm with 1 cm sample thicknesses. This provided a weaker platinum-rich sample at 151 cm as well as a weak osmium-rich sample at the same depth. This latter measurement, HC17_44, appears to be the last one they made. Until then, no osmium was detected by Sun et al. (2020) at the YDB.
Later in the same section we read;
HEA: The ammonium and nitrate spike appears in the ice core even higher corresponding to about 50 years later than the YDB.
This concerns the ammonium ion spike plotted in Petaev et al. (2013). It misrepresents the data on which it is based from Mayewski et al. (1993), as described in Sweatman (2021). The correct data is shown in Sweatman (2021), also provided below in Figure 15 .
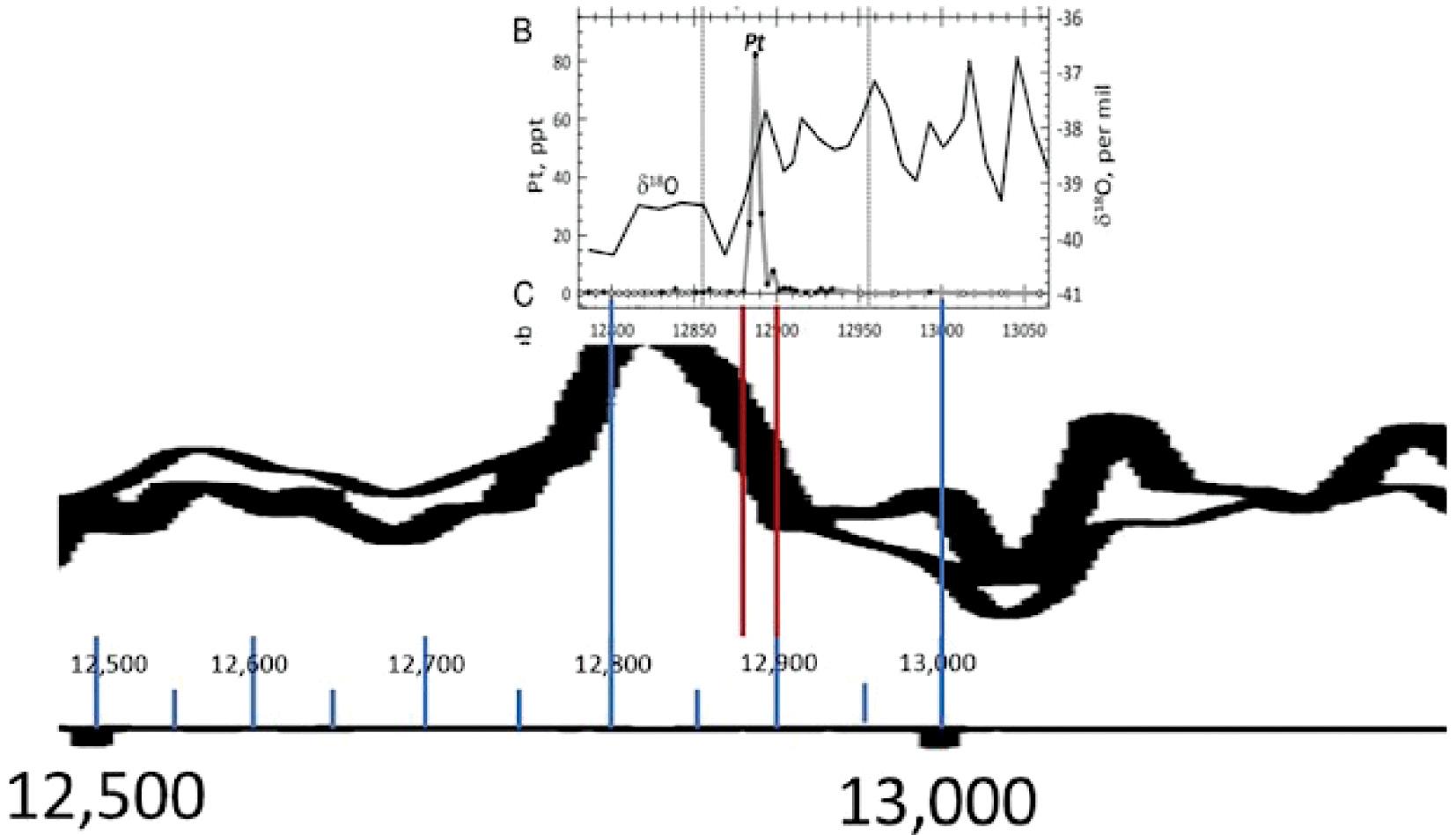
Part of Figure 3 from Sweatman (2021) showing the strong correlation between the platinum spike and an ammonium bump in the GISP2 ice core.
The inset plot shows the timing of the GISP2 platinum signal versus the oxygen isotope signal in the GISP2 ice core. Underneath is the GISP2 ammonium ion signal in Mayewski et al. (1993), which is misleadingly plotted as a single spike at its center by Petaev et al. (2013). The vertical lines tie these two plots together. All this data is from the same ice core, and therefore cannot be misaligned. Clearly, the onset of these signals are synchronous within the resolution of the data (red guide lines). This was emphasized by Sweatman (2021) but is ignored by HEA.
However, higher resolution data for the ammonium anomaly is given in Wolbach et al (2018a), shown above in Figure 16. This shows the platinum spike and sudden drop in temperature (signaled by the oxygen isotope trace) coincide with a steadily increasing ammonium signal in the GISP2 ice core. The ammonium signal is very noisy, indicating ammonium is transported to Greenland from accumulations elsewhere by fluctuating weather and storms. HEA ignore this data too.
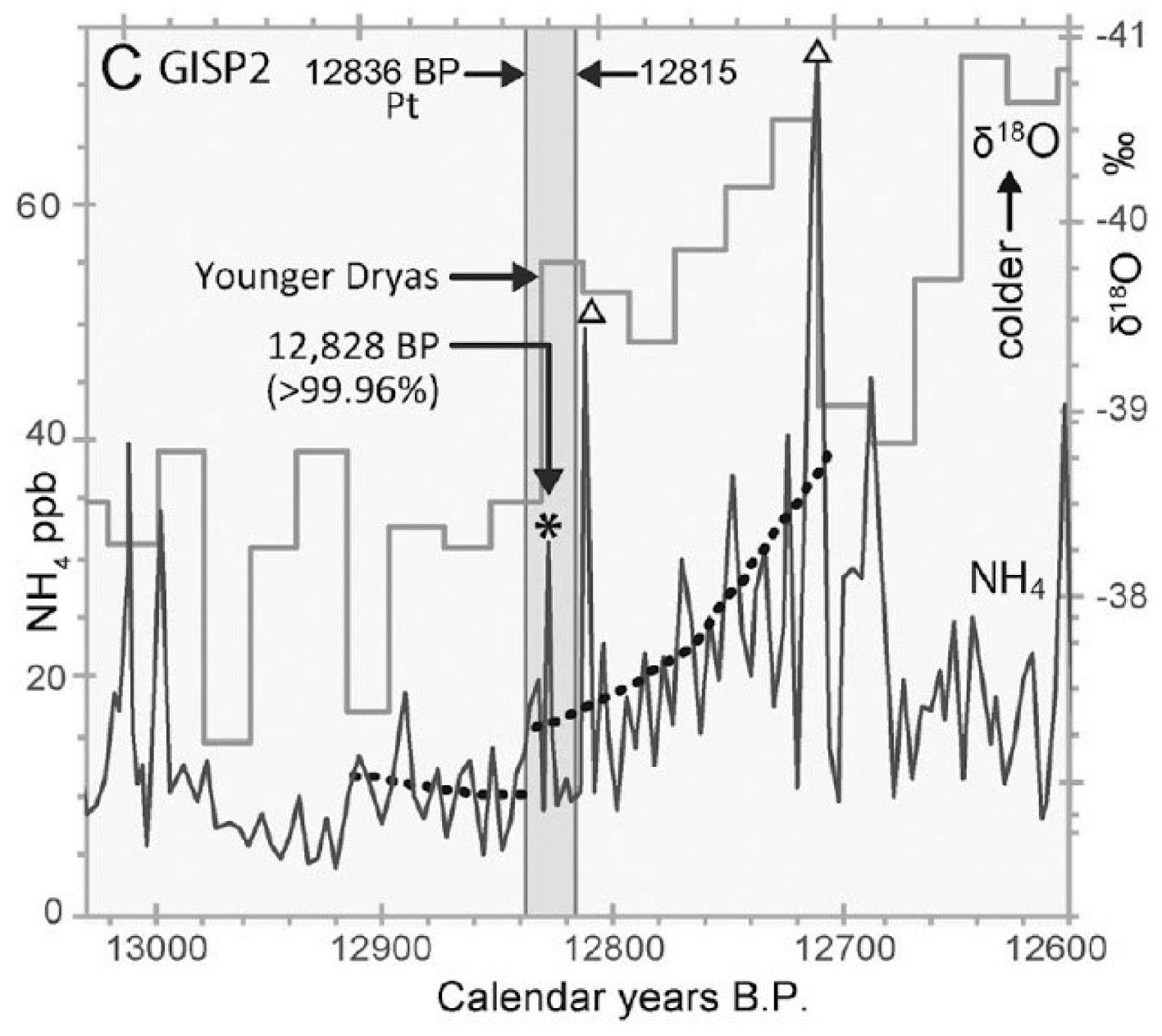
High resolution ammonium data from the YD period plotted in Figure 3C of Wolbach et al. (2018a).
HEA: This suggests three independent events with three different causes.
Clearly, the beginning of the YD ammonium ion ‘bump’ coincides with the platinum signal in the GISP2 ice core, as shown above in Figures 15 and 16. How the YD onset is defined is a matter of contention and open to interpretation. But a coincident event remains a possibility.
7.6. Summary of Section 7
These extracts from several sections of HEA and our comments above clearly show that HEA repeatedly distort the facts to make their case. In particular, they;
Repeatedly ignore the YDIH scenario update in Wolbach et al. (2018a) despite discussing it in earlier work. Instead, they muddy the water by assigning a wide variety of sources equal status regarding the favored YDIH impact scenario, regardless of their context. This is highly misleading.
Argue that coherent catastrophism is a ‘speculative hypothesis that is unsupported by observational data and inconsistent with the cratering record’. And yet, it is the working model for formation of the Taurid meteor stream among cometary scientists. HEA even remark that coherent catastrophism is contradicted by the latest 2019 observational campaign of the Taurid resonant swarm. But they neglect to mention that this campaign did not take place as planned and no papers have been published about it, and the existence of the Taurid resonant swarm was already confirmed in earlier observational campaigns.
Frequently demand evidence for ground impacts when these impacts are not a requirement of the YDIH. Moreover, they state that microspherules cannot be used alone to diagnose an ET impact, yet co-authors of HEA have published a review article (French and Koeberl (2010)) that contradicts that view. Furthermore, it is only through selectively quoting from this source that HEA avoid this contradiction.
Conflate discussion of the nanodiamond evidence in Daulton et al. (2010) with later papers by Daulton et al. (2017). In fact, it is abundantly clear that the nanodiamond evidence in Daulton et al. (2010) is fundamentally flawed since their samples are not from where they claim and, in any case, they cannot be expected to contain nanodiamonds. Daulton et al. (2010) should be retracted.
Use Sun et al. (2020) to critique the platinum evidence in the YDIH. Yet Sun et al. (2020) appear to have omitted data in their report that would have corroborated the YDIH. This needs to be explained by Sun et al. (2020).
Misrepresent ammonium ion data from the GISP2 ice core, essentially ignoring Sweatman’s (2021) observations that this data is consistent with the YDIH.
8. Instances of Derision in HEA
It is generally accepted among scholars that research articles in peer-reviewed scholarly journals should be respectful and free of derisory comments. This includes proscription of ad hominem remarks which are aimed at the person rather than the scientific evidence. Moreover, since Earth Science Reviews is an Elsevier journal it is subject to Elsevier’s publishing policy which states;
“It is important to lay down standards of expected ethical behavior by all parties involved in the act of publishing: the author, the journal editor, the peer reviewer, the publisher and the society for society-owned or sponsored journals. This includes all parties treating each other with respect and dignity and without discrimination, harassment, bullying or retaliation. (Publishing Ethics | Elsevier Policy, n.d.)”
It is therefore disappointing to see many derisory remarks in HEA. Below, we compile quotes from HEA where they repeatedly make derisory remarks that appear to violate the above Elsevier policy. Our comments are indented in italics.
HEA’s Section 1: Introduction
HEA: Firestone et al. (2006, 2007) were the first publications to gain wide attention, in part due to an AGU symposium in 2007 that drew considerable attention from the news media. The book is based on fanciful speculation and demonstrates a remarkable lack of understanding of the archaeological and stratigraphic data discussed. It contains many examples of misleading or blatantly untrue statements (noted throughout this review) and was described by Morrison (2010) as “pseudoscience.”
This is untrue and is an inaccurate reflection of Morrison’s statement. In any case, Morrison’s opinion concerns a speculative book published before the YDIH was proposed in a peer-reviewed journal. Use of the word “pseudoscience” is derisory and disrespectful and there is no justification for this remark in a peer-reviewed article. We note, however, that HEA rely heavily on Meltzer et al. (2014) which claims to be scientific, but omits confidence intervals for its age-depth models which would have nullified its conclusions. Measurements without confidence intervals are not scientific.
HEA: Claiming evidence where none exists and providing misleading citations may be accidental, but when conducted repeatedly, it becomes negligent and undermines scientific advancement as well as the credibility of science itself.
This comment comes very close to an unfounded accusation of scientific misconduct. It is therefore in violation of Elsevier’s policy above.
Many lines of evidence for the YDIH have been reproduced by independent research groups. Failures at reproduction by YDIH opponents are often due to their own faulty experimental protocols.
HEA: The Proceedings of the National Academy of Sciences “contributed review” system for National Academy members (e.g., Aldous, 2014), as in the case of Firestone et al. (2007) and Kennett et al. (2009), is at least partially responsible. The “pal reviews” (as some refer to them) were significantly curtailed in 2010, in part due to the YDIH controversy.
This is another implied suggestion of scientific misconduct and strenuously disputed. It says in effect that the Firestone et al. (2007) article could not have been published without bias on the part of the peer reviewers. This is pure speculation for which there is not a shred of evidence and clearly does not apply to work published since 2010 which has repeatedly corroborated the YDIH. It is also a disparaging ad hominem attack on YDIH proponents and appears to violate Elsevier’s publication policy above.
HEA: Section 5.2: Pseudoarchaeological Divined Date of the Impact Event
No justification by HEA is provided for use of the derisory phrases “pseudoarchaeological” and “divined” in this subtitle whatsoever. In fact, the subject of this section, Sweatman and Tsikritsis (2017a), is published in a peer-reviewed archaeological journal and HEA offer no critical analysis of this work at all. Indeed, HEA’s criticism of Sweatman and Tsikritsis (2017a) appears to rest heavily on quotes from Notroff et al. (2017), but no explanation is provided by HEA as to why these quotes from Notroff et al. (2017), who disagree with Sweatman and Tsikritsis (2017a), must mean that Sweatman and Tsikritsis (2017a) is pseudoarchaeological. After all, archaeologists disagree frequently about the interpretation of archaeological sites. For example, Banning (2011) also disputed early interpretations of Gobekli Tepe’s enclosures. Should we consider his work pseudoarchaeological too? Nor do HEA cite the rebuttal by Sweatman and Tsikritsis (2017b) to Notroff et al. (2017) which HEA should know exists because it is provided in the same pdf download as Notroff et al. (2017) by the journal. In their rebuttal, Sweatman and Tsikritsis (2017b) convincingly rebut Notroff et al.’s (2017) criticisms. Indeed, recent work by Sweatman (2024) published after HEA provides strong support for Sweatman and Tsikritsis’ (2017a) astronomical interpretation.
HEA’s Section 5.3: Deficient Dating of YDIH Sites
HEA: LeCompte et al. (2018, p156) complain that YDIH critics “do not use rigorous dating methods…”
HEA’s Section 6: Misinterpreted black mats
HEA: Authors common to YDIH-proponent papers with opposing black mat interpretations appear confused and lacking in credibility.
This is an ad hominem, and completely unjustified. Section 6 of HEA mainly consists of wordplay around the characterization of the YD black mat, the YDB, and how they relate. Contrary to their claims, proponents of the YDIH have a very good understanding of these concepts, and their claims regarding them have often been misrepresented by critics. HEA’s confusion possibly stems from the fact that characteristics of the YD black mat vary between sites. This is expected because of local geography and the proximity of each black mat site to different local impact events. Thus, HEA’s description of “opposing interpretations” simply reflects the varying nature of the YD black mat in different locations.
HEA’s Section 7: Multifarious YDB impact scenarios
HEA: Proponents of the YDIH claim that purported Pt anomalies at the YDB (Section 11) are strong evidence of meteoritic material and an ET event (e.g., Moore et al., 2017; Sweatman, 2021; Powell, 2020, 2022) and ardently cite measurements of Greenland ice by Petaev et al. (2013a).
HEA’s Section 10: Purported YDIH evidence of impact: spherules/microspherules
HEA: Powell (2022, p 14) concludes, “The simplest explanation is again that Firestone et al. sampled the YDB at Topper while Surovell et al. did not.” The condescending argument about procedural errors is an after-the-fact explanation of inconvenient data.
The word “condescending” is a pejorative and should not be included in any scientific report. Powell (2022) is merely providing a possible reason why Surovell et al. (2009) failed to find any microspherules at all at Topper, whereas others have done so and photographed them.
HEA’s Section 13: Fanciful YDIH indicators, abandoned claims, and mislaid or missing evidence
HEA: The early claims include interpretations of geomorphic records, stratigraphic sections, and geochemical data that were speculative and sometimes contradictory. Most of this alleged evidence disappeared from the current YDIH literature with no comment, but its highly speculative nature certainly reflects on the credibility of the authors that were involved.
Questioning the “credibility” of YDIH authors is a disparaging ad hominem, and therefore should not appear in any peer-reviewed scientific report. There can be many reasons why some lines of evidence are pursued over others that have nothing to do with the credibility or competence of the researchers involved, and in general they require no justification. Changing one’s mind in the face of new evidence is not only good science, it is necessary.
HEA’s Section 13.1: Carolina Bays and High Plains playas
HEA: There is no evidence for playa formation at ~12.9 cal ka BP. Claiming that a paper states the opposite conclusion to make a point about purported impacts represents scientific malfeasance.
This implied accusation of scientific malfeasance is made without any supporting evidence whatsoever and is therefore completely unjustified and defamatory. Researchers can often make mistakes or misunderstand the evidence or simply speculate within the bounds of uncertainty. The same can be said of their accusers. This does not represent malfeasance. However, implied accusations of malfeasance where none has occurred corrupts the scientific record.
HEA: Section 13.3 More pseudoscience (fringe) evidence and conjecture
The label “pseudoscience” is derisory and should never be used in a scientific report. It is generally reserved for claims that are inconsistent with established norms of science, such as perpetual motion, mind-reading and fairies. The definition is sometimes extended to work that claims to be scientific but uses non-scientific methods.
The term “fringe” is used to describe research with little support among the scientific community, often because it is a new idea. Therefore, all new scientific ideas begin as fringe ideas. In fact, science requires a steady supply of fringe ideas; without fringe ideas science dies. Therefore, use of the word “fringe” as a label in a scientific paper is itself anti-science, derisory and irrelevant.
Use of both these terms is prevalent on social media sites, such as Wikipedia. However, it is crucially important that the norms of social media do not become the norms of scientific publishing.
Many quotes and sources provided by HEA in this section are from speculative works that are not peer-reviewed or intended for a scientific audience. Few of the cited sources are peer-reviewed papers relevant to this debate. Those that are pertinent are certainly not pseudoscience.
HEA’s Section 13.4: Mislaid Greenland ice expedition
HEA: In August 2009, members of the group returned to the location where samples were collected one year earlier (Heidari, 2010; Allen West email to Mark Boslough, September 25, 2010). They collected and processed samples seeking more evidence but never published any further findings. Thus, it appears that the report of hexagonal nanodiamonds in Greenland Ice by Kurbatov et al. (2010) has not been replicated despite at least one attempt.
The implied accusation here is that negative results for hexagonal nanodiamonds were not published. This unsubstantiated claim is completely unjustified and defamatory. There can be many reasons why scientific studies are not published that have nothing to do with negative results and HEA provide no evidence whatsoever that negative results were obtained but not published on that basis.
HEA’s Section 13.5: Mislaid contrary evidence
HEA: Proponents of the YDIH fail to report negative or conflicting results, for example, dating of Carolina Bays (Section 13.1), fullerenes with ET helium (Section 13.2), ‘nanodiamonds’ in Greenland ice (Section 13.4), and ‘nanodiamonds’ at Bull Creek (Section 12.6). More troublesome is that YDIH proponents continue to report original results as valid even after failed attempts to reproduce those results.
This statement clearly implies scientific malpractice where there is none and is therefore completely unjustified and defamatory. Regarding the dating of Carolina Bays, fullerenes with ET helium, and nanodiamonds in Greenland ice, HEA provide no evidence for any malpractice by YDIH proponents whatsoever. Regarding the the Bull Creek nanodiamonds, the apparent discrepancy is demonstrated in section 6.3 to be a misunderstanding by HEA. While Bement et al. (2014) mis-plotted Kennet et al.’s (2009) data and might have mis-stated units, these errors do not affect their conclusions which strongly support the YDIH. HEA, on the other hand, appear to have mistreated Kennett et al.’s (2009) nanodiamond data by shifting it upwards by one full sampling interval (see Section 5.7 of HEA in our Section 6 above).
Regarding failed attempts to reproduce YDIH evidence by YDIH opponents, in most cases this is clearly caused by faulty methods used by them. For example, it is clear that Daulton et al. (2010) failed to find nanodiamonds at Arlington Canyon because they took samples that are not expected to contain nanodiamonds from the wrong location, while claiming they were taken from the correct location. Moreover, Meltzer et al. (2014), upon which HEA rely heavily, omitted confidence intervals in their age-depth models. Had they done so correctly, their conclusions would have been nullified. And Sun et al. (2020) seem to have omitted important data from Hall’s Cave that would have supported the YDIH. Meanwhile, Jorgeson et al. (2020, 2022) fail to understand the limitations of their own models. Furthermore, HEA mistreat the data for nanodiamonds at the Bull Creek YDB while simultaneously claiming this evidence contradicts the YDIH and is ignored by YDIH proponents. HEA even appear to selectively quote from French and Koeberl (2010). The fact is, the evidence and arguments that YDIH opponents rely upon usually has obvious flaws. HEA disregard or misunderstand these flaws and instead imply scientific malpractice on behalf of YDIH proponents. We recommend HEA should pause, take note of the obvious methodological errors of work that opposes the YDIH, and take responsibility for their own misunderstandings of the YDIH. Accusations or implications of malpractice should only be made where the evidence is abundantly clear. False or unsupported accusations are defamatory and a corruption of the scientific record, especially where the fault clearly lies with the accuser.
HEA: Section 15. Unparalleled promotion of the YDIH outside of scientific literature
The entirety of Section 15 of HEA is an ad hominem attack. It contains no evidence or arguments about the physical data and focuses instead solely on the extra-curricular activities of YDIH proponents. It is completely inappropriate for a scientific report and should never have passed peer or editorial review.
HEA’s Section 17: Conclusions
HEA: The YDIH evolved directly from pseudoscience.
HEA: The first widely read paper on the YDIH (Firestone et al., 2007) had a range of serious weaknesses, reviewed here and by others. Although the 2007 paper did not contain all the pseudoscience of its predecessors, it was nevertheless plagued with poor age control, faulty assumptions, and misstatements of fact.
The accusation of pseudoscience relating to Firestone et al. (2007) is completely unjustified and derisory. We remind HEA of the many misconceptions, misunderstandings and faulty methods, highlighted in this rebuttal, that are used by opponents of the YDIH in their work and that HEA rely upon.
8.1. Summary of Section 8
We have provided numerous examples of disparagement by HEA directed against YDIH proponents, including ad hominem remarks and unfounded accusations of scientific malpractice. Such comments contravene Elsevier’s own policy covering publication ethics and therefore none of them should have passed peer review.
Powell (2024) shows that such comments are not isolated to HEA but instead form part of a pattern of increasing disparagement by YDIH opponents directed against YDIH proponents. Powell (2024) argues that this rhetoric aims to suppress research into the YDIH and therefore HEA, along with several earlier works by YDIH opponents, can be labelled as “pseudoskepticism”. Indeed, the title of HEA’s work which claims “comprehensive refutation” without providing any refuting arguments is part of this pattern.