Introduction
Positron emission tomography (PET) is a powerful noninvasive imaging tool for phenotyping patients at risk of or with known coronary artery disease (CAD) that has been evolving in the past 30 years. One of the key advantages over other imaging modalities is its unique ability to quantify physiologic processes, including myocardial blood flow (in milliliters per minute per gram of myocardium), metabolism, and cardiac receptors, and its high sensitivity for delineating and quantifying molecular targets in vivo using targeted imaging probes. The discussion that follows will review the emerging applications of cardiac PET/computed tomography (CT) in diagnosis and management of CAD.
Technical Considerations
Several technical advantages account for the improved image quality and diagnostic ability of PET compared with conventional radionuclide imaging, including (1) routine measured (depth-independent) attenuation correction (with either 68Ge sources for PET-only systems or CT for hybrid PET/CT scanners), which decreases the occurrence of false positives and thus increases specificity, (2) high spatial and contrast resolution (heart-to-background ratio) that allows improved detection of small perfusion defects, thereby decreasing the occurrence of false negatives and increasing sensitivity, and (3) high temporal resolution that allows fast dynamic imaging of tracer kinetics, which makes absolute quantification of myocardial perfusion (in milliliters per minute per gram of tissue) possible. In addition, the use of short-lived radiopharmaceuticals allows fast, sequential assessment of regional myocardial perfusion (e.g., rest and stress), thereby increasing laboratory efficiency and patient throughput.
Multiparametric PET/CT
All modern PET systems are now combined with a CT scanner into a hybrid PET/CT camera. Each component of the integrated system provides unique information for the evaluation of patients with known or suspected CAD as described later. Figure 1 illustrates current state-of-the-art cardiac PET/CT protocols for the evaluation of known or suspected CAD. As discussed later, each of the components of the multiparametric imaging protocol provide complementary information regarding diagnosis and risk stratification.
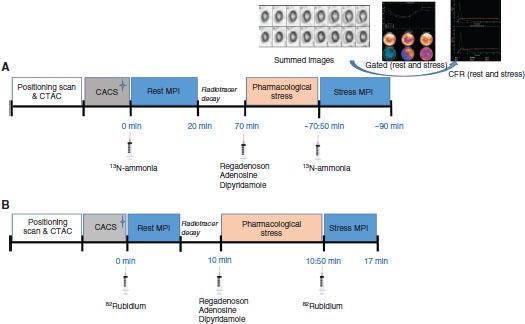
Multiparametric Positron Emission Tomography/Computed Tomography (CT) Protocol Using (A) 13N-ammonia and (B) Rubidium-82 as Blood Flow Tracers
CACS, coronary artery calcium scan (optional); CFR, coronary flow reserve; CTAC, CT attenuation correction scan; MPI, myocardial perfusion imaging.
Emission Scans
Because of the short physical half-life of PET radiopharmaceuticals for the assessment of myocardial perfusion, approximately the same dose is injected for both rest and stress myocardial perfusion studies. The injected doses are adjusted according to the size of the patient and the type of PET data acquisition (i.e., 2-D mode vs. 3-D mode). List mode imaging has become standard practice with modern PET technology, which allows a comprehensive multiparametric examination (Figure 1), which includes the assessment of myocardial perfusion, left ventricular ejection fraction (LVEF) and left ventricular (LV) volumes, and quantitative coronary blood flow and flow reserve in the same setting. In select patients with severe LV dysfunction, metabolic imaging with fluorodeoxyglucose (FDG) is used to assess myocardial viability.
CT Scans
Low-dose CT scans are used for positioning patients in the scanner field of view. A low-dose nongated CT scan covering the heart region (transmission scan) is then used for correction of the inhomogeneities of radiotracer distribution in the PET images caused by overlapping soft tissue (e.g., breast, diaphragm). This postprocessing step is known as attenuation correction and is mandatory for cardiac PET. In select patients, a gated CT scan may be obtained to calculate a coronary artery calcium (CAC) score (Agatston score). Finally, it is also possible to obtain a coronary CT angiogram immediately following the assessment of myocardial perfusion and/or metabolism.
PET/CT Imaging Markers Used in Diagnostic and Prognostic Evaluation of CAD
Quantification of Myocardial Ischemia and Scar
Regional myocardial perfusion is usually assessed by semiquantitative visual analysis of the rest and stress images. The segmental scores are then summed to give global scores (the summed stress score reflects the extent and severity of ischemia plus scar; the summed rest score reflects the extent and transmurality of scar; the summed difference score reflects the extent and severity of ischemia) that reflect the total burden of ischemia and scar in the left ventricle. These global scores are typically expressed as a percentage of the LV mass, which is estimated by division of the corresponding global score by the total maximum score possible [17 segments×4 (worst score in a given segment)=68]. The semiquantitative (visual) and quantitative scores of ischemia and scar reflect the extent and severity of CAD, and are linearly related to the risk of adverse cardiovascular events. Large and severe perfusion defects (typically involving more than 10% of the LV mass), and those involving multiple coronary territories are associated with higher clinical risk. Therefore the quantification of ischemia and scar is useful in guiding patient treatment, especially the need for revascularization. The presence of transient LV dilatation during stress imaging (so-called transient ischemic dilatation) is an ancillary marker of risk that reflects extensive subendocardial ischemia from multivessel obstructive coronary stenosis and/or microvascular dysfunction (Figure 2).
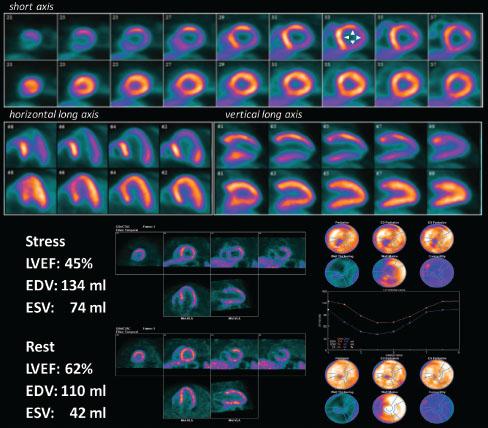
High-risk Myocardial Perfusion Positron Emission Tomography/Computed Tomography Scan.
Stress and rest myocardial perfusion study demonstrating all the features of a high-risk scan, including transient ischemic dilatation with stress (arrowheads), multiple territorial perfusion defects including more than 10% of the left ventricular mass, and a drop in left ventricular ejection fraction (LVEF) with stress associated with enlargement of the end-systolic volume (ESV). EDV, end-diastolic volume.
Quantification of Myocardial Blood Flow and Coronary Flow Reserve
Myocardial blood flow (in milliliters per minute per gram of myocardium) and coronary flow reserve (CFR; defined as the ratio between peak stress and rest myocardial blood flow) are important physiologic parameters that can be measured by routine postprocessing of myocardial perfusion PET images (Figure 3). These absolute measurements of tissue perfusion are accurate and reproducible. CFR estimates provide a measure of the integrated effects of epicardial coronary stenoses, diffuse atherosclerosis and vessel remodeling, and microvascular dysfunction on myocardial perfusion, and, as such, the value obtained is a more sensitive measure of myocardial ischemia. In the setting of increased oxygen demand such as during exercise, a reduced CFR can upset the supply-demand relationship and lead to myocardial ischemia, subclinical LV dysfunction (diastolic and systolic), symptoms, and death. As discussed later, these measurements of CFR have important diagnostic and prognostic implications in the evaluation and treatment of patients with known or suspected CAD.
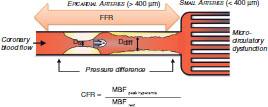
Conceptual Schematic of Coronary Flow Reserve (CFR) Measurements.
CFR is a quantitative measure of coronary vascular dysfunction that integrates the hemodynamic effects of focal, diffuse, and small-vessel coronary artery disease on myocardial tissue perfusion. CFR is calculated as the ratio of hyperemic to rest absolute myocardial blood flow (MBF). In contrast, measurements of fractional flow reserve (FFR) integrate only the effects of focal epicardial stenosis and diffuse atherosclerosis.
Assessment of Myocardial Viability
Myocardial perfusion imaging (MPI) and metabolic imaging are commonly used to evaluate the patient with ischemic LV dysfunction, especially when the question of revascularization is being considered. Radionuclide imaging provides important quantitative information, including (1) myocardial infarct size, (2) extent of stunning and hibernating myocardium, (3) magnitude of inducible myocardial ischemia, and (4) LV function and volumes. Metabolic imaging with PET has been extensively validated and is commonly used for assessment of myocardial viability. 18FDG is used to assess regional myocardial glucose utilization (an index of tissue viability) and the images obtained are compared with perfusion images to define metabolic abnormalities associated with infarction and hibernation. Reduced perfusion and increased FDG uptake at rest (so-called perfusion-FDG mismatch) identifies areas of viable but hibernating myocardium, whereas regions showing both reduced perfusion and reduced FDG uptake at rest (so-called perfusion-FDG match) are consistent with myocardial scar (Figure 4). As discussed later, these metabolic patterns are powerful markers of risk, and have important implications for the selection of patients for revascularization.

Myocardial Viability Positron Emission Tomography (PET) Patterns.
The top panel shows an example of a patient with a large perfusion defect throughout the anterior and apical walls with concordant reduction in myocardial glucose uptake (PET match). The bottom panel shows an example of a patient with a large perfusion defect of moderate intensity throughout the anterior and apical walls with a relative increase in myocardial glucose uptake (PET mismatch). FDG, fluorodeoxyglucose (metabolic tracer); Rb-82, eubidium-82 (flow tracer). Reproduced with permission from Di Carli et al. [1].
Quantification of LV Function and Volumes
The acquisition of ECG-gated myocardial perfusion images allows quantification of regional and global systolic function, and LV volumes. With PET, ECG-gated images are typically collected at rest and during stress. Rest LVEF measurements are helpful to define the patient's risk after myocardial infarction. A drop in LVEF during pharmacologic stress can be helpful to identify high-risk patients.
Quantification of Coronary Artery Calcifications
The widespread use of integrated PET/CT scanners allows the routine quantification of CAC scores, which as discussed later improves risk assessment especially in patients with suspected CAD. Transmission CT scans used for attenuation correction can also be used for a semiquantitative assessment of coronary artery calcifications.
Cardiac PET/CT in Diagnosis, Risk Stratification, and Management of CAD
Diagnosis of CAD
Recent meta-analyses [2, 3] and a prospective European multicenter study [4] have confirmed the high sensitivity and diagnostic accuracy of PET MPI for the detection of significant angiographic stenosis. In addition, a more recent meta-analysis using fractional flow reserve (FFR) as a gold standard for flow-limiting CAD demonstrated high sensitivity, specificity, negative predictive value, and positive predictive value for PET [5] (Figure 5). In this analysis, PET's performance in excluding abnormal FFR was comparable to that of cardiac MRI and CT, and superior to that of both single photon emission (SPECT) and echocardiography. As described earlier, one additional advantage of PET is that it allows routine quantification of myocardial blood flow and CFR. These quantitative measures of myocardial perfusion increase the sensitivity and the negative predictive value of PET for ruling out high-risk angiographic CAD [6–10].

Pooled Estimates of Diagnostic Accuracy of Myocardial Perfusion Imaging with Single Photon Emission Computed Tomography, Echocardiography, MRI, and Positron Emission Tomography.
AUC, area under the receiver operating characteristic curve; NLR, negative likelihood ratio; PLR, positive likelihood ratio. Data obtained from Takx et al. [5].
Risk Stratification of Known or Suspected CAD with PET MPI
The power of radionuclide MPI (including PET) for risk stratification is based on the fact that the major determinants of prognosis in patients with CAD are readily available from gated MPI. These include the amount of myocardial scar, the extent and severity of stress-induced ischemia, and measurements of LV size (i.e., volumes) and LVEF. Optimal risk stratification is based on the concept that the risk associated with a normal study is sufficiently low that revascularization will not further improve patient outcomes. Hence catheterization is an unlikely option after testing. Conversely, patients with abnormal stress imaging results are at greater risk of adverse events and thus are potential candidates for intervention, and the magnitude of their risk is related to the extent and severity of the imaging abnormalities.
There is growing and consistent evidence documenting the value of PET MPI in risk stratification of CAD. In general, normal PET MPI findings are associated with a very low risk of adverse cardiac events, and the risk increases linearly with the extent and severity of perfusion abnormalities. Multiple single-center studies and a large multicenter registry have demonstrated that the concept of progressive risk stratification initially shown with SPECT MPI abnormalities is also applicable to PET studies. In a large multicenter observational registry of 7061 patients with known or suspected CAD from four sites (median follow-up 2.2 years), stress PET results added incremental value over preimaging data for the prediction of cardiac death. Risk-adjusted analyses revealed that mildly, moderately, and severely abnormal findings were associated with a stepwise increase in clinical risk (Figure 6). The study showed that for each 10% increase in ischemic and scarred myocardium, the risk of cardiac death increased by 34% and 57% respectively. Patients with severely abnormal stress PET MPI findings had a nearly fivefold higher risk of cardiac death compared with patients with normal PET MPI findings, even after other clinical risk markers had been accounting for. Further, PET MPI results enhanced net reclassification improvement [11].

Positron Emission Tomography Myocardial Perfusion Imaging for Risk Stratification.
Adjusted Kaplan-Meier survival curves and hazard ratios by extent of myocardium with abnormal perfusion. CD, cardiac deaths; CI, confidence interval; HR, hazard ratio; Reproduced with permission from Dorbala et al. [11].
A subsequent study from this registry addressed the question of sex-related prognostic differences after stress PET and demonstrated that PET MPI yielded incremental prognostic value in both sexes, which, in turn, was associated with similar magnitudes of risk in men and women. Finally, a complementary study from this registry showed that normal PET MPI findings were associated with an excellent prognosis with very low annual cardiac death rates in normal (0.38%), overweight (0.43%), and obese (0.15%) patients. As shown for other cohorts, risk increased with increasing degree of perfusion abnormalities in PET MPI [12].
Ancillary Markers of Clinical Risk
As for conventional radionuclide perfusion imaging, the use of ECG gating is an integral part of the PET MPI examination, thereby allowing quantification of regional and global LV systolic function and volumes, and improved risk stratification. Indeed, the presence of normal LVEF and wall motion identifies a lower-risk cohort even in the setting of perfusion abnormalities [13]. Conversely, the presence of LV dilatation (transient or fixed), wall motion abnormalities either at rest or after stress, and reduced LVEF have all been well described as markers of increased patient risk.
A unique aspect of PET MPI resides in its ability to quantify LVEF at rest and during peak stress, similarly to stress echocardiography. In normal individuals, LVEF increases during peak vasodilator stress [14]. In patients with obstructive CAD, however, the change in LVEF from the baseline to peak stress (so-called LVEF reserve) is inversely related to the extent of obstructive angiographic CAD [14]. Patients with obstructive multivessel or left main artery disease show a frank drop in LVEF during peak stress even in the absence of apparent perfusion defects. In contrast, those without significant CAD or with mild angiographic CAD show a normal increase in LVEF [14–16]. More importantly, the lack of augmentation in LVEF from the baseline to peak stress identifies patients at increased risk of adverse cardiac events and provides incremental risk stratification [14].
The presence of transient LV dilatation and increased right ventricular tracer uptake with stress are also markers of diffuse myocardial ischemia from obstructive multivessel CAD and/or microvascular dysfunction and are associated with increased risk (Figure 2).
Coronary Flow Reserve
In addition to the diagnostic utility of quantitative measures of myocardial blood flow discussed already, CFR is also emerging as a powerful quantitative prognostic imaging marker of clinical cardiovascular risk. From a pathophysiologic viewpoint, CFR provides a robust and reproducible clinical measure of the integrated hemodynamic effects of epicardial CAD, diffuse atherosclerosis, vessel remodeling, and microvascular dysfunction on myocardial tissue perfusion. Consequently, it is a more sensitive measure of myocardial ischemia and overall vascular health that provides unique information about clinical risk. The available data thus far suggest that CFR measurements by PET can distinguish patients at high risk of serious adverse events, including cardiac death [10, 17–19]. A severely reduced CFR (<1.5) was associated with a nearly sixfold increased risk of cardiac death compared with a relatively preserved CFR (>2.0) (Figure 7), even after adjustment for differences in clinical risk, the extent and severity of ischemia and scar, and LVEF, and led to clinically meaningful risk reclassification of approximately 50% of intermediate-risk patients [17]. Importantly, an abnormal CFR identified increased risk of cardiac death even among those patients with normal scans by semiquantitative visual analysis. As discussed, the noninvasive PET measure of CFR has been able to improve risk classification, especially among high-risk cohorts (e.g., diabetic patients, non-ST-elevation myocardial infarction patients, in patients with chronic renal impairment, and in those with high CAC scores) [20–22]. For example, a reduced global CFR was independently associated with higher rates of cardiac and all-cause death in a large cohort of patients with and without diabetes mellitus. Compared with diabetic patients with preserved CFR, those with reduced CFR experienced substantially higher cardiac mortality [20] (Figures 8 and 9). Importantly, diabetic patients without known CAD and with impaired CFR experienced a rate of cardiac death comparable to, and possibly higher than, that for nondiabetic patients with known CAD. These observations have implications for the classification of diabetes mellitus as a coronary disease risk equivalent [23]. Specifically, only among diabetic patients with impaired vascular function is the prognosis comparable to that for nondiabetic patients with known CAD. Thus differing levels of vascular health among previously studied cohorts may account for inconsistencies in the relative mortality rates of diabetic patients without CAD and nondiabetic patients with CAD [24–26]. Thus the ability to assess CFR appears to permit a level of risk assessment beyond that achieved previously, with the potential to incorporate vascular/endothelial status into routine patient investigations. In patients with impaired CFR and severely obstructive CAD, preliminary data support a possible benefit from revascularization by coronary artery bypass grafting (Figure 10) [21].

Coronary Flow Reserve (CFR) and Risk Stratification.
Cumulative incidence of cardiac death by tertiles of CFR. CD, cardiac deaths; HR, hazard ratio. Reproduced with permission from Murthy et al. [17].

Examples of Risk Reclassification by Coronary Flow Reserve (CFR).
Top panel: A 58-year-old man with hypertension and diabetes evaluated for atypical chest pain with positron emission tomography (PET) myocardial perfusion imaging (MPI). The study shows normal myocardial perfusion and CFR>2 in all coronary territories. Lower panel: A 63-year-old man with hypertension, diabetes, and high cholesterol level evaluated for dyspnea. PET MPI shows normal myocardial perfusion but CFR is severely reduced in all coronary territories, consistent with severe microvascular dysfunction. LAD, left anterior descending artery; LCX, left circumflex artery; LV, left ventricle; RCA, right coronary artery.

Coronary Flow Reserve (CFR) Reclassifies Risk of Cardiac Death in Diabetic Patients.
Adjusted annualized cardiac mortality rates among patients stratified by the presence or absence of diabetes mellitus (DM) and coronary artery disease (CAD). EF, ejection fraction; LVEF, left ventricular ejection fraction; MPI, myocardial perfusion imaging; NI, no ischemia. Reproduced with permission from Murthy et al. [20].

Coronary Flow Reserve (CF), Revascularization, and Outcomes.
Adjusted annualized rates of cardiovascular death and heart failure admission among patients referred for coronary angiography stratified by CFR and an early revascularization strategy (coronary artery bypass grafting [CABG], percutaneous coronary intervention [PCI], or neither). No difference in event rates was seen in patients with high global CFR (left panel), regardless of the revascularization strategy. In patients with low CFR (right panel), those who underwent CABG had lower event rates than those who underwent PCI or no revascularization. Reproduced with permission from Taqueti et al. [21].
This ability to quantify CFR extends the potential for evaluation of risk to those patients in the past not considered to be candidates for testing. Even in patients without CAD and with normal LV function, after adjustment for multiple confounders, impaired CFR was associated with both the occurrence of a positive troponin test and with major downstream adverse events [21]. With use of PET-derived CFR, the prognostic impact of coronary microvascular dysfunction was assessed in 405 men and 813 women who were identified as having no prior CAD as well as no perfusion abnormalities in their PET MPI studies [27]. Coronary microvascular dysfunction, defined as a CFR of less than 2.0 in these patients, was a frequent finding in both men and women (51% and 54% respectively). Patients were followed up for a median of 1.3 years for the composite end point of cardiac death, nonfatal myocardial infarction, late revascularization, and hospitalization for heart failure. In both men and women, CFR added incremental prognostic value (hazard ratio 0.80 [95% confidence interval 0.75–0.86] per 10% increase in CFR) and appropriately reclassified patients with respect to risk, as assessed by significant net reclassification improvement (Figure 11). These findings suggest that coronary microvascular dysfunction is a widespread finding and that work is needed to identify its putative role as a therapeutic target.

Microvascular Dysfunction (MCD) in Males and Females Without Obstructive Coronary Artery Disease.
Distribution of coronary flow reserve (CFR) by sex. The pie charts demonstrate that more than 50% of males and females show reduced CFR, consistent with MCD. The histogram (top right) shows similar severity of MCD regardless of sex. The major adverse cardiac event (MACE) rate (lower right) was higher among patients with reduced CFR regardless of sex. Reproduced with permission from Murthy et al. [27].
CAC and Coronary Artery Plaque Characterization
As all modern PET scanners are combined with CT scanners, we are beginning to understand the potential complementary roles between structure and function for diagnosis and management of CAD. There is growing evidence that CAC scores are generally predictive of a higher likelihood of ischemia (reflecting obstructive CAD) on MPI [28–31]. However, the relationship between the extent and the severity of coronary calcification and measures of myocardial ischemia reflecting obstructive CAD is quite modest [32, 33]. Importantly, CAC scores less than 400, especially in symptomatic patients with intermediate likelihood of CAD, have limited sensitivity and negative predictive value to exclude CAD especially in young individuals and women [31, 34, 35].
The integration of CAC scores and ischemia information also offers a unique opportunity to refine the prognostic value of myocardial perfusion PET. The CAC score, reflecting the anatomic extent of atherosclerosis [36], offers an opportunity to improve the conventional models for risk assessment using nuclear imaging alone (especially in patients with normal perfusion). For example, the presence of extensive coronary artery calcifications in a patient with normal PET MPI findings identifies patients at higher risk of adverse cardiac events as compared with patients with normal MPI findings and no or mild coronary artery calcifications [31] (Figure 12).

Risk Stratification by Positron Emission Tomography (PET) Myocardial Perfusion Imaging (MPI) and Coronary Artery Calcium (CAC) Scores.
Panels A and B show examples of normal PET MPI findings in two patients at intermediate clinical risk, suggesting no evidence of flow-limiting coronary artery disease and presumably similarly low posttest clinical risk. However, the addition of the CAC score reveals extensive atherosclerosis in the patient in panel B, and suggests higher clinical risk. The bar chart demonstrates a differential major adverse cardiac event rate for patients with normal PET MPI findings stratified by the CAC score. Reproduced with permission from Schenker et al. [31].
With the addition of intravenous contrast material, coronary CT angiography further expands the role of hybrid PET/CT for diagnosis and management of CAD. There is evidence that hybrid imaging may offer superior diagnostic information with regard to identification of the culprit vessel [37–40]. Coronary CT angiography enhances the sensitivity for detection of multivessel CAD, while MPI increases the specificity and positive predictive value for delineation of flow-limiting coronary artery stenosis. The addition of coronary CT angiography is also quite helpful to help exclude severe coronary artery obstruction among patients with severely reduced CFR [8].
Myocardial Metabolism for Delineation of Myocardial Viability
PET has an established role in the evaluation of myocardial ischemia and viability in patients with ischemic heart failure. Several studies using different radionuclide approaches including PET have shown that the gain in global LVEF after revascularization is related to the magnitude of viable myocardium assessed preoperatively. These data demonstrate that clinically meaningful changes in global LV function can be expected after revascularization only in patients with relatively large areas of hibernating and/or stunned myocardium (~20% of the LV mass). In addition to the obtaining of information regarding viability, ischemia assessment can also be performed during the same examination. The use of viability imaging also plays a role in determining the size and extent of scar in planning optimal implantable cardiac defibrillator/cardiac resynchronization therapy lead position in patients with ischemic cardiomyopathy. Studies have shown that CRT-D (implantable cardiac defibrillators enabled with cardiac resynchronization therapy) devices are more successful when the lead position is in an area of viable myocardium, with improved LV systolic function and outcomes [41].
More importantly, there are consistent data from single-center, observational studies demonstrating that the presence of ischemic, viable myocardium among patients with severe LV dysfunction identifies patients at higher clinical risk, and that prompt revascularization in select patients is associated with improved LV function, abatement of symptoms, and increased survival as compared with medical therapy alone [42]. The randomized PARR-2 clinical trial demonstrated that PET-guided decisions regarding revascularization could also help improve clinical outcomes following revascularization if treatment decisions adhere to imaging recommendations [43].
Conclusions
PET/CT is a powerful tool for translational research that offers unique quantitative insights into molecular processes in vivo. Hybrid PET/CT is rapidly advancing our ability to image in great detail the structure and function of the heart and vasculature. By providing concurrent quantitative information about myocardial blood flow and metabolism with coronary and cardiac anatomy, PET/CT offers the opportunity for a comprehensive noninvasive evaluation of the consequences of atherosclerosis in the coronary arteries and the myocardium. This integrated platform for assessing anatomy and biology expands the potential for translating molecularly targeted imaging into humans.