Severe acute respiratory syndrome coronavirus 2 (SARS-CoV-2), the pathogen responsible for coronavirus disease 2019 (COVID-19), has caused a global pandemic since its emergence in Wuhan, China in late 2019 [1]. As of January 5, 2022, it has caused more than 296 million infections leading to more than 5.4 million fatalities [2]. As a positive single-stranded RNA virus whose polymerase lacks proofreading capability, SARS-CoV-2 frequently mutates during viral replication, thus generating numerous viral variants with altered viral infectivity, transmission, and disease severity [3]. The original Wuhan isolate sequence of the spike glycoprotein on SARS-CoV-2, which binds the cellular receptor angiotensin converting enzyme 2 (ACE2), was used for vaccine development. New mutations in the spike gene have continually emerged in variants over time. This Commentary (i) summarizes the key variants and their spike mutations, and (ii) discusses their implications in viral replication, transmission, and immune evasion.
DOMINANT SARS-COV-2 VARIANTS
Among all SARS-CoV-2 strains, some notable variants have fitness advantages and have outcompeted other variants during viral evolution. The first dominant spike (S) protein D614G substitution increases viral replication in the human upper respiratory tract; this mutant rapidly replaced nearly all prior SARS-CoV-2 strains from June 2020 onward [4]. Subsequently, the Alpha variant (B.1.1.7 lineage) first reported in the United Kingdom and quickly spread to many parts of the world, owing to its increased binding affinity for human ACE2 receptor [5–7]. Next, the Delta variant (B.1.617.2 lineage) first reported in India in October 2020, spread rapidly, and displaced the Alpha variants worldwide [8–10]. Recently, the heavily mutated Omicron variant, first detected in South Africa in November 2021, has explosively spread to many countries and led to global Omicron surges [11–13]. According to previous studies on known mutations and clinical observations, the Omicron variant is transmitted much more rapidly than the Delta variant, with a case doubling time as short as 1.5–3 days. In addition, Omicron can escape many monoclonal antibodies and evade vaccine-elicited neutralization [14–16]. Furthermore, infection with previous non-Omicron variants does not appear to elicit robust neutralization against Omicron [17]. However, booster vaccination (e.g., a third dose of the Pfizer/BioNTech vaccine or a booster dose of the Moderna vaccine) elicits good neutralization against Omicron [18], thus supporting a booster vaccination strategy. The durability of the protective immunity of booster vaccination against Omicron remains to be determined. Omicron’s high transmissibility and immune evasion have enabled it to become the new dominant SARS-CoV-2 variant. More than 1 million cases in a single day have been recorded in the United States (Fig 1).
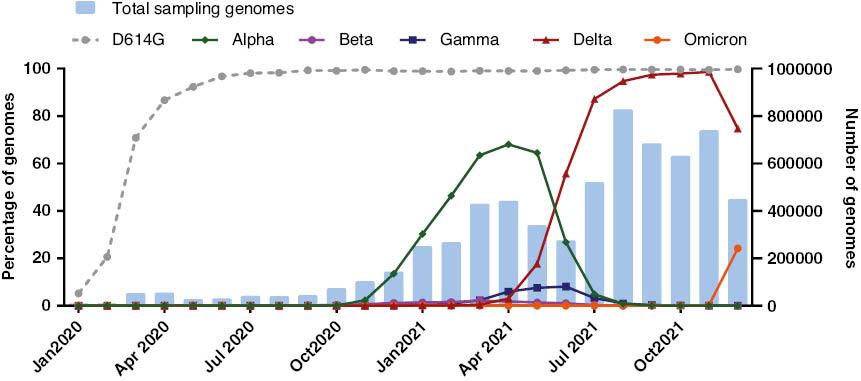
Frequencies of the D614G, Alpha, Beta, Gamma, Delta, and Omicron lineages over time in all genomic SARS-CoV-2 sequences.
Blue bars represent the total numbers of SARS-CoV-2 genomes. Colored lines indicate the percentages of different lineages in the total SATS-CoV-2 genome. All data were sourced from the GISAID database from January 2020 to December 2021.
NOTABLE MUTATIONS IN THE S PROTEIN
The trimeric S glycoprotein of coronaviruses is the major surface protein present on the viral envelope [19]. Mature S protein, formed through cleavage by furin and transmembrane serine protease 2 (TMPRSS2), mediates viral binding to the ACE2 receptor, entry, and immune escape. Thus, S protein is considered the key determinant of viral infectivity and transmissibility [20]. The D614G substitution is the first dominant mutation that occurred in the S protein. Elevated quantities of D614G virus have been detected in the respiratory tracts in both patients and animal models, thus indicating this mutant’s higher infectivity and transmissibility [21,22]. Another mutation, N501Y, located at the receptor-binding domain in the S protein, has been demonstrated to be the most important amino acid substitution in the Alpha variant. The N501Y substitution markedly increases the binding affinity to the human ACE2 receptor, thus leading to rapid infection in the upper respiratory tract and higher viral transmission [7,23]. The Delta variant has the spike mutation P681R, which is located at a furin-cleavage site in the S protein. Experimental results in human primary airway epithelial culture and animal models have demonstrated that the P681R substitution enhances viral replication and infection, possibly through increasing the furin cleavage of the full-length S protein into S1 and S2 subunits [10,24]. Many other amino acid changes in the S protein, including S13I, L18F, 69–70 deletion, W152C, K417N/T, N439K, N440K, L452R, Y453F, S477G/N/R, E484K/Q/P, S494P, and H655Y, have been reported to decrease vaccinated serum neutralization and monoclonal-antibody inhibition, and/or increase viral infection and transmission (Table 1). The Omicron variant contains more than 30 mutations in the S protein, several of which are present in other variants of concern, including Alpha, Beta, Gamma, and Delta [39]. Some of these mutations, including 69–70 deletion, K417N, N440K, S477N, E484A, N501Y, D614G, H655Y, and P681H, have been well studied and are known to enhance viral infectivity, transmissibility, and immune escape, thus leading to high concern regarding the pandemic’s potential severity resulting from ongoing Omicron surges.
Notable mutations increasing SARS-CoV-2 infectivity, transmissibility, and immune escape.
Mutations | Locations | Representative Lineages | Functions | References |
---|---|---|---|---|
S13I | N-terminal domain | B.1.427 | Immune escape | [25] |
L18F | N-terminal domain | Beta, Gamma | Immune escape | [26] |
del69–70 | N-terminal domain | Alpha, Omicron* | Immune escape/increased transmissibility | [27] |
W152C | N-terminal domain | B.1.427 | Immune escape | [25] |
K417N/T | Receptor-binding domain | Beta, Gamma, Omicron | Attenuated biding affinity to ACE2/immune escape | [28] |
N439K | Receptor-binding domain | AV.1 | Increased binding affinity to ACE2/immune escape | [29] |
N440K | Receptor-binding domain | Omicron | Increased infectivity | [30] |
L452R | Receptor-binding domain | Delta | Immune escape | [31–33] |
Y453F | Receptor-binding domain | Delta | Immune escape | [34] |
S477G/N/R | Receptor-binding domain | Omicron | Immune escape | [35] |
E484K/Q/P | Receptor-binding domain | Beta, Gamma, Delta, Eta, Lota, Kappa, Mu, Omicron | Immune escape | [31,32] |
S494P | Receptor-binding domain | Alpha | Immune escape | [36,37] |
N501Y | Receptor-binding domain | Alpha, Beta, Gamma, Mu, Omicron | Increased infection and transmissibility | [7,28] |
D614G | The C-terminal of S1 domain | All lineages | Increased infectivity | [22] |
H655Y | Near S1/S2 cleavage site | Gamma, Omicron | Immune escape | [38] |
P681H/R | Near S1/S2 cleavage site | Alpha, Kappa, Mu, Omicron | Increased cleavage efficiency | [10] |
*Omicron is underlined to indicate multiple mutations and deletions with respect to other variants.
NEUTRALIZATION OF SARS-COV-2 VARIANTS AND VACCINE STRATEGIES
Several COVID-19 vaccines have been approved and used for immunization globally to develop herd immunity against COVID-19 [40]. The Pfizer/BioNTech BNT162b2 nucleoside-modified mRNA vaccine is one of the most common vaccines, which has been widely used in North America and Europe [41]. Because of the high mutation frequency of SARS-CoV-2 S protein, some newly emerged variants have diminished susceptibility to neutralization by antibodies generated by vaccination or natural infection. Several approaches have been used to measure the neutralization sensitivity of variants to human sera, including pseudotype virus for expression of SARS-CoV-2 S protein, clinical viral isolates, and chimeric SARS-CoV-2 bearing the S protein from different variants [13,14,42]. Among these approaches, the use of chimeric SARS-CoV-2 bearing variant S has two major advantages. First, this approach does not require waiting for the isolation of clinical viral strains; as soon as the S sequence is available, the variant S sequence can be synthesized and engineered into the original SARS-CoV-2 backbone [43]. Second, in this approach, in contrast to the pseudovirus approach, the chimeric virus is an authentic SARS-CoV-2. The 50% plaque-reduction neutralization titer against various recombinant viruses can be easily tested and accurately compared among all variants. The Alpha variants have higher infection and transmission efficiency, but the neutralization titers are approximately equivalent to those of the WT strain [42]. The D614G and representative Delta variants exhibit modestly lower neutralization titers than the WT virus [44,45]. Unfortunately, two doses of the Pfizer vaccine are insufficient to induce robust antibody neutralization against the Omicron variant [46]. The above studies were all performed on the same set of human sera collected 2 or 4 weeks after the administration of two doses of the Pfizer vaccine (Fig 2). Many other studies have also demonstrated that the neutralization titers of infected and vaccinated people are significantly lower against Omicron than the WT virus [17,18]. However, as described above, a third dose of the Pfizer vaccine increases the magnitude and breadth of neutralization, thus leading to robust neutralization against the Omicron variant [18,46,47]. To date, the neutralization level has been found to remain robust as long as 4 months after the third dose of the Pfizer vaccine; however, the durability of neutralization beyond 4 months after the third dose remains to be determined [46]. These results support a two-pronged vaccine strategy against Omicron and other newly emerged variants, involving (i) booster vaccination with the currently approved safe vaccines and (ii) modification of the vaccine S sequences to match those of Omicron and new variants. The mRNA vaccine technology allows for rapid modification of the S sequence. Real-world vaccine effectiveness data and laboratory studies are needed to guide the implementation of this two-pronged vaccine strategy.
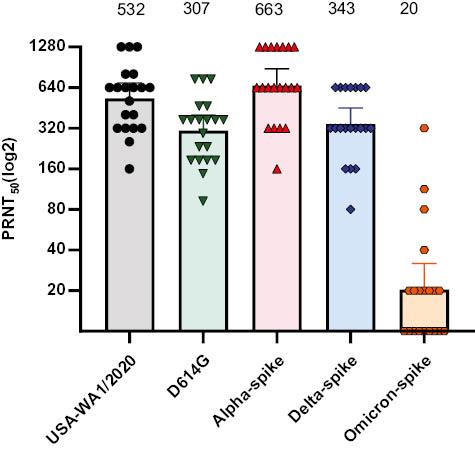
Serum neutralization titers of different lineages of SARS-CoV-2.
The D614G mutation or genes encoding S protein from different lineages of SARS-CoV-2 were engineered into the USA-WA1/2020 backbone. The 50% plaque-reduction neutralization testing (PRNT50) for 20 samples obtained from 15 trial participants after administration of the second dose of the BNT162b2 vaccine is shown. Each data point represents the geometric mean PRNT50 obtained with a serum sample against the indicated virus. The heights of bars and the numbers over the bars indicate geometric mean titers. The error bars indicate 95% confidence intervals.
Two years have elapsed since the onset of the COVID-19 pandemic, and various SARS-CoV-2 variants, in turns, have dominated viral transmission and surges. The current Omicron surge may not be the last. Although some variants have become able to escape immune protection from vaccination and/or natural infection [43–45,48], compelling evidence demonstrates that vaccination minimizes the risk of severe disease, and lowers the rates of hospitalization and death [49,50]. Thus, mass immunization and administration of booster shots with highly effective and safe vaccines, together with mask wearing and social distancing, will continue to be the most effective strategies to finally end the COVID-19 pandemic.