INTRODUCTION
Hard ticks (Acari: Ixodidae) are obligate blood-sucking parasitic arthropods that are vectors for a wide range of zoonoses, such as tick-borne encephalitis; Lyme borreliosis; and Anaplasma, Coxiella, Ehrlichia and Rickettsia and Babesia infections [1–3]. Tick-transmitted infections often occur in people working in forested areas or farmers engaged in animal husbandry [4]. Therefore, hard ticks are considered a relatively greater threat to animal and human health. Hyalomma marginatum, a species of hard ticks, is a known arthropod vector of several viruses, such as Thogoto, Dhori, Crimean-Congo hemorrhagic fever, and West Nile viruses [5], as well as bacteria, such as Rickettsia aeschlimannii, which is associated with spotted fever infection in humans [6].
To date, 119 tick species in ten genera have been reported in China, including 100 species of hard ticks [7,8]. The increasing cases of tick-borne diseases in humans, including Q fever [9], spotted fever [10], ehrlichiosis [11], anaplasmosis [12], brucellosis [8], tick-borne encephalitis [13], and babesiosis [14], have been reported in ticks in this region. Tibet, located in the southwestern of Qinghai-Tibet Plateau in China, supports the survival of several microorganisms that are adapted to the harsh environment of the plateau. Because of the harsh natural environment, animal husbandry and livestock are cornerstone industries in Tibet. Haemaphysalis tibetensis [15], Boophilus microplus [16], and Dermacentor spp. [17,18] have been found in Tibet, and several tick-borne pathogens (TBPs), such as Anaplasma marginale, Anaplasma ovis, GRD spirochetes, and Ehrlichia spp. have been reported in ticks in this region [12]. However, limited knowledge exists regarding the distribution of Hy. marginatum and TBPs in Hy. marginatum in Tibet.
In this study, TBPs in Hy. marginatum feeding on yaks in Tibet were analyzed through metagenomic next-generation sequencing (mNGS) followed by genus/group-specific nested polymerase chain reaction (PCR). The results of this study provide broader information on the microorganisms maintained by Hy. marginatum in the region.
METHODS
Study design
A two-stage protocol was used in the present study: tick species were first identified through morphological characterization and molecular biology methods; mNGS was then used for TBP identification in individual ticks and was followed by genus/group-specific nested PCR (Fig 1).
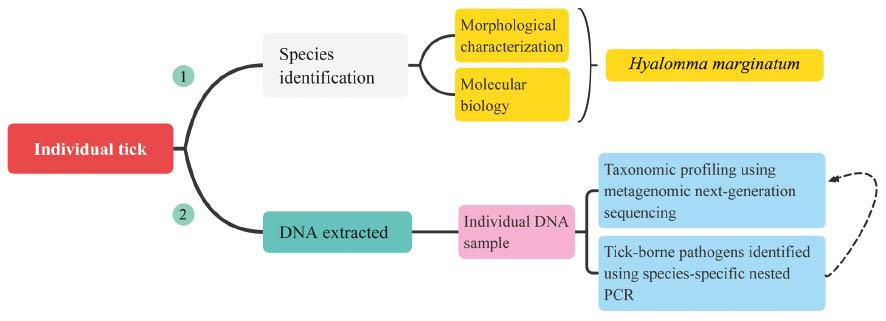
Study workflow. Morphological characterization and molecular biology methods were performed for identification of tick species, and metagenomic next-generation sequencing followed by genus/group-specific nested PCR was subsequently used for tick-borne pathogen identification in individual ticks.
Tick collection
All ticks collected were feeding on yaks from Chagyab County in Tibet in October of 2019 (Fig 2). The ears, groin, tail, abdomen, and neck regions of yaks of different ages and sexes were examined for the presence of ticks, and all visible ticks were collected with forceps. Tick species were identified on the basis of morphological characterization and molecular biology methods, according to the sequence of the mitochondrial cytochrome c oxidase I (COI) gene, as described in previously [19].
DNA extraction
First, ticks were surface sterilized with 75% ethanol twice, followed by phosphate-buffered saline twice to remove environmental contaminants. Then individual ticks were homogenized in phosphate-buffered saline with MagNA Lyser Green Beads (Roche, Mannheim, Germany), and DNA was extracted with a QIAamp® Fast DNA Tissue Kit (Qiagen, Dusseldorf, Germany) according to the manufacturer’s instructions. Deionized water was added as an extraction control. Finally, each extracted DNA sample was eluted in 100 μL deionized water and stored at -20°C until further analysis.
Metagenomic sequencing and taxonomy prediction
Individual DNA samples were sequenced on the Illumina HiSeq platform with paired-end 150-bp reads by Novogene (Beijing, China). Reads with low quality bases (quality threshold value ≤ 38) above a certain length (more than 40 bp) and/or with more than 10 bp of “N” bases were removed. Reads with more than 15 bp overlap with the adapter were also removed. Reads of host origin were finally filtered. Consequently, clean data were obtained.
The clean reads were assembled and analyzed with SOAPdenovo [20]. The scaffolds were broken at N into the scaftigs, and the scaftigs (<500 bp) were filtered [21]. The scaftigs (≥500 bp) were predicted according to the open reading frames by MetaGeneMark [22,23], and CD-HIT was used to remove redundancy and obtain the initial unique gene catalog [24,25]. For determination of gene abundance, the reads were realigned with the gene catalog with Bowtie 2. Only genes with two or more mapped reads were deemed to be present in a sample [26]. The relative abundance of each gene in each sample was calculated according to the number of mapped reads and the length of the gene [27–29].
Unigenes were aligned to the NR database (https://www.ncbi.nlm.nih.gov/) of NCBI with DIAMOND [30]. The aligned results of each gene with e value ≤ the smallest e value × 10 were retained [26] and then processed with the Lowest Common Ancestor-based algorithm implemented in MEGAN to ensure the species annotation information of sequences [31]. The final results contained the number of genes and the abundance information for each sample, and the relative abundance of each taxonomic group was calculated by addition of the relative abundance of genes annotated to the same feature [21,23,32].
Polymerase chain reaction (PCR)
Genus/group-specific nested PCR was performed to confirm the presence of TBPs in individual ticks. The PCR primers for spotted fever group rickettsia (SFGR) [33,34], Anaplasma spp. and Ehrlichia spp. [35] are presented in Table 1. Briefly, 2 μL DNA of sample was subjected to the initial PCR run, and 2 μL of PCR product from the first round was subjected to a second round of PCR. All PCR amplifications were performed with PrimeSTAR® HS (Premix) (TaKaRa, Beijing, China) and a PCR System 9700 (Applied Biosystems, GeneAmp®, USA). The nested PCR products were separated electrophoretically in 1.5% agarose gel, and positive amplicons were sequenced.
Target genes and primer sequences used for PCR
Pathogen | Target gene | Primer name | Sequence (5′-3′) |
---|---|---|---|
SFGR | gltA | CS2d | ATGACCAATGAAAATAATAAT |
CSEndr | CTTATACTCTCTATGTACA | ||
RpCS.877p | GGGGACCTGCTCACGGCGG | ||
RpCS.1258n | ATTGCAAAAAGTACAGTGAACA | ||
ompA | Rr190.70p | ATGGCGAATATTTCTCCAAAA | |
Rr190.602n | AGTGCAGCATTCGCTCCCCCT | ||
190.70-38s1 | AAAACCGCTTTATTCACC | ||
190.602-384r1 | GGCAACAAGTTACCTCCT | ||
17 kDa | R17122 | CAGAGTGCTATGGAACAAACAAGG | |
R17500 | CTTGCCATTGCCCATCAGGTTG | ||
TZ15 | TTCTCAATTCGGTAAGGGC | ||
TZ16 | ATATTGACCAGTGCTATTTC | ||
ompB | BG1-21 | GGCAATTAATATCGCTGACGG | |
BG2-20 | GCATCTGCACTAGCACTTTC | ||
Gene D | D1F | ATGAGTAAAGACGGTAACCT | |
D928R | AAGCTATTGCGTCATCTCCG | ||
Anaplasma spp. | 16S rRNA | Eh-out1 | TTGAGAGTTTGATCCTGGCTCAGAACG |
Ehrlichia spp. | Eh-out2 | CACCTCTACACTAGGAATTCCGCTATC | |
Eh-gs1 | GTAATAACTGTATAATCCCTG | ||
Eh-gs2 | GTACCGTCATTATCTTCCCTA |
Phylogenetic analysis
The obtained DNA sequences were compared with the reference sequences in GenBank with the NCBI-BLAST server (http://blast.ncbi.nlm.nih.gov/blast.cgi), and multiple sequences were aligned with ClustalW with default parameters in MEGA 7.0. The phylogenetic tree of outer membrane protein A (ompA), citrate synthase (gltA), ompB, gene D, and 17kDa for SFGR was constructed with the maximum likelihood method on the basis of the T92+G model, T92 model, T92 model, T92 model, and k2 model, with 1000 bootstrap replicates in MEGA 7.0 [36,37].
RESULTS
Taxonomic classification
All 14 adult ticks were identified as Hy. marginatum, according to morphological identification and species-specific PCR targeting the COI gene. Thirteen individual DNA samples were successfully analyzed with metagenomic sequencing. Sequencing yielded between 52597 and 58706 million reads per sample library, all of which had high quality (Clean_Q20 > 95%) (Table 2). The construction of the metagenomic library of sample 1.9 failed.
Pooling strategies for metagenomic next-generation sequencing
Sample ID | InsertSize (bp) | SeqStrategy | RawData (MB) | CleanData (MB) | Clean_Q20 | Clean_GC (%) |
---|---|---|---|---|---|---|
CYP1.1 | 350 | (150:150) | 57,988.81 | 56,715.91 | 96.95 | 47.63 |
CYP1.2 | 350 | (150:150) | 55,630.90 | 54,744.23 | 96.59 | 47.42 |
CYP1.3 | 350 | (150:150) | 55,016.44 | 53,340.28 | 96.61 | 48.19 |
CYP1.4 | 350 | (150:150) | 56,170.53 | 54,486.79 | 96.91 | 47.62 |
CYP1.5 | 350 | (150:150) | 56,013.06 | 54,700.57 | 97.19 | 47.41 |
CYP1.6 | 350 | (150:150) | 54,318.37 | 52,685.77 | 96.93 | 48 |
CYP1.7 | 350 | (150:150) | 52,597.53 | 51,261.33 | 96.22 | 47.61 |
CYP1.8 | 350 | (150:150) | 53,026.99 | 51,275.89 | 96.31 | 47.28 |
CYP1.10 | 350 | (150:150) | 55,921.43 | 54,575.12 | 96.36 | 46.72 |
CYP1.11 | 350 | (150:150) | 54,589.54 | 52,202.90 | 96.94 | 47.31 |
CYP1.12 | 350 | (150:150) | 57,403.60 | 55,920.88 | 96.31 | 47.4 |
CYP1.13 | 350 | (150:150) | 57,792.73 | 56,846.56 | 96.34 | 47.75 |
CYP1.14 | 350 | (150:150) | 58,706.93 | 56,559.08 | 97.37 | 46.97 |
Metagenomic sequencing was performed to analyze the microbial community. A total of 276419 core genes were predicted to be common to all 13 tick samples, thus indicating their similar microbial community (Fig 3). The presence of Rickettsia, Anaplasma, and Ehrlichia at the genus level in individual tick samples was identified according to the taxonomic profiles. Rickettsia spp. was most abundant in all samples, followed by Ehrlichia spp. In addition, Anaplasma spp. was detected in samples CYP1.3, CYP1.4, and CYP1.10, but with lower abundance than that of Ehrlichia spp. In addition, Staphylococcus spp. were the most abundant microorganisms in all samples, followed by Escherichia spp. (Fig 4A).
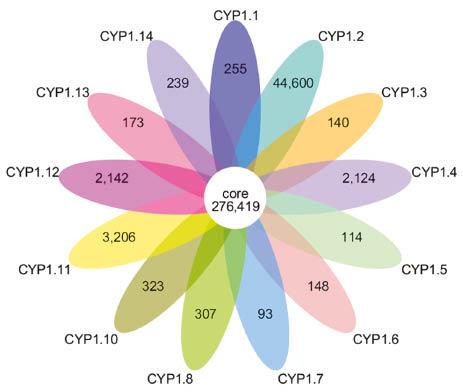
Total numbers of shared and unique core genes of individual ticks. CYP1.1 to CYP1.14, individual DNA samples of Hy. marginatum for metagenomic next-generation sequencing.
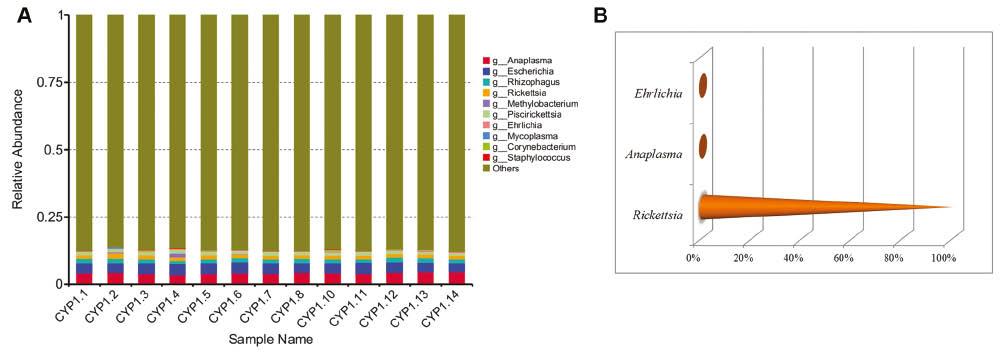
Analysis of the microbial community in Hyalomma marginatum. The relative abundance of the potential top ten bacteria at the genus level was analyzed with metagenomic next-generation sequencing (A), and then the tick-borne pathogens maintained in individual ticks were confirmed by genus/group-specific nested PCR (B). CYP1.1 to CYP1.14, individual DNA samples of Hy. marginatum.
Prevalence of Rickettsia in individual ticks
The presence of Rickettsia, Anaplasma, and Ehrlichia was identified in individual ticks with mNGS, and the identification of TBPs in individual ticks was confirmed by genus/group-specific PCR combined with sequencing.
Consequently, detection of Anaplasma spp. and Ehrlichia spp. yielded no positive results with nested PCR targeting the 16S rRNA gene. Only Rickettsia spp. detection was positive with nested PCR targeting the ompA, gltA, ompB, gene D, and 17kDa genes, and positive amplification of these two genes was found in all 13 tick samples (13/13, 100%) (Fig 4B). The obtained sequences for each gene for SFGR from all 13 tick samples were 99.61% and 100% identical to R. aeschlimannii, respectively.
Phylogenetic analysis
According to phylogenetic analysis based on the partial sequences of ompA, gltA, ompB, gene D, and 17kDa genes, R. aeschlimannii, identified in Hy. marginatum in the present study, clustered with other R. aeschlimannii subspecies and was most closely associated with R. aeschlimannii stavropol (DQ235777), which had been isolated from Hy. marginatum in Russia (Figs 5 and 6).
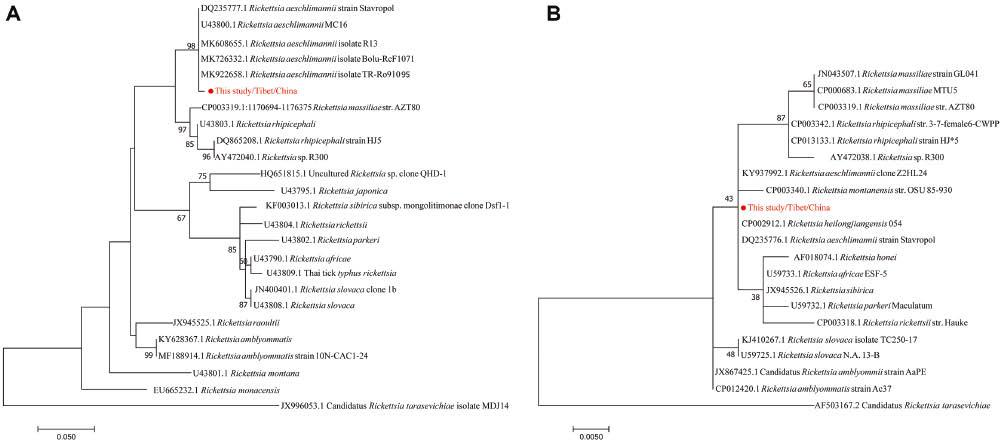
Phylogenetic analysis based on the sequences of the ompA and gltA genes of Rickettsia aeschlimannii. The obtained sequences in the present study are indicated with red dots. Multiple sequences were aligned with the ClustalW tool in MEGA 7.0. Phylogenetic analysis of ompA (258 bp) (A) and gltA (285 bp) (B) of R. aeschlimannii was performed with the maximum likelihood method based on the T92+G model and T92 model with 1000 bootstrap replicates in MEGA 7.0.
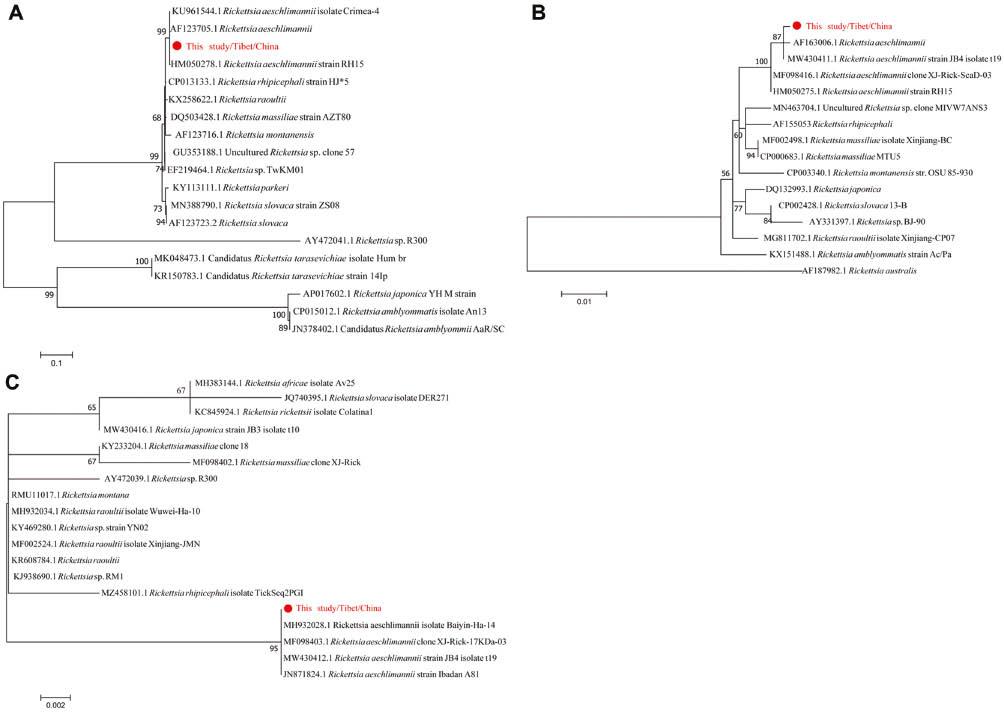
Phylogenetic analysis based on the sequences of the ompB, gene D, and 17 kDa genes of Rickettsia aeschlimannii. The obtained sequences in the present study are indicated with red dots. Multiple sequences were aligned with the ClustalW tool in MEGA 7.0. Phylogenetic analysis of ompB (564 bp) (A), gene D (729 bp) (B), and 17 kDa (163 bp) (C) of R. aeschlimannii was performed with the maximum likelihood method based on the T92 model, T92 model, and k2 model with 1000 bootstrap replicates in MEGA 7.0.
DISCUSSION
Recently, increasing attention has been paid to the distribution of ticks and TBPs worldwide, and this knowledge can aid in the prevention and control of tick-borne diseases. Although a variety of TBPs have been identified in ticks in China, knowledge regarding the distribution of Hy. marginatum and TBPs in Tibet remains limited. In the present study, mNGS combined with nested PCR was applied to survey TBPs in Hy. marginatum feeding on yaks in Tibet, China.
mNGS has been successfully used to identify known and/or unknown microorganisms in arthropods [38,39], thus enabling analysis of microorganism diversity. By mNGS, the most abundant bacteria identified in ticks include Rickettsia spp., Coxiella spp., Francisella spp., and “Candidatus Midichloria mitochondrii” [40]. Ravi et al. have applied mNGS to analyze the microbiomes in hard ticks collected in Palestine and detected high levels of important TBPs, including Coxiella spp., Rickettsia spp., and A. ovis, and generated a genome sequence of a canine parvovirus [41]. Beyond these bacteria, environmental and skin-associated bacteria, such as Pseudomonas, Acinetobacter, Enterobacter, and Stenotrophomonas, are common in hard ticks [42,43]. Bacteria such as Staphylococcus and Escherichia might have been predominant in the present study.
The results of mNGS in the present study revealed the presence of Rickettsia, Anaplasma, and Ehrlichia in Hy. marginatum. However, only Rickettsia spp. was positively detected in genus/group-specific nested PCR. After sequence comparison, R. aeschlimannii was found in all 13 Hy. marginatum. The results of genus/group-specific nested PCRs were partially consistent with those of mNGS, possibly because less abundant species might not have been sensitively amplified with PCR when highly abundant sequences were overrepresented [40]. In addition, Coxiella spp., Babesia spp., and Borrelia spp. were often detected in ticks. Thus each tick was detected by genus/group-specific nested PCR in the present study, and no positive amplicons were observed (data not shown).
Hyalomma spp. (e.g., Hy. marginatum) are mainly endemic to southern and eastern Europe [44], and these ticks also have been reported in birds in countries where these ticks are not autochthonous [45]. One possible explanation is that the larvae and nymphs of Hyalomma spp. may be transported passively by migratory birds from southern Europe to their breeding areas in the northern hemisphere [44,45]. Hy. marginatum, a species of Hyalomma spp., is distributed primarily in northern Africa, southern and eastern Europe, the Middle East and several parts of Asia [5,46]. To date, Hy. marginatum has been found in Inner Mongolia, Gansu, and Xinjiang in China [10,47,48], thus indicating that this tick is not autochthonous in China. To our knowledge, this is the first report of Hy. marginatum in Tibet, thus indicating its increasing geographical distribution in China.
R. aeschlimannii belongs to SFGR and was first detected in Hy. marginatum in Morocco in 1997 [49]. A clinical case of infection caused by this pathogen was first reported in 2002 [6]. Symptoms of R. aeschlimannii infection in humans are highly similar to those of Mediterranean spotted fever caused by R. conorii and may be associated with liver dysfunction [50]. In China, R. aeschlimannii has been detected in Rhipicephalus turanicus [51], Hy. asiaticum, [10,47], Hy. marginatum [10], and Haemaphysalis punctata [52]. Yang et al. have reported a case of R. aeschlimannii infection in a woman from Xingjiang, and this pathogen was also detected in Hy. asiaticum around the patient’s residence [53]. In the present study, R. aeschlimannii was detected in all Hy. marginatum, thereby indicating its dominant prevalence in these ticks; this finding may be relevant to the health of humans who may have spotted fever after being bitten by these ticks.
This study has several limitations. Although the researchers went to Zogang County, Chamdo County, and Chagyab County, and verified nearly 100 yaks for tick collection, the high altitude (>4000 meters) and low environmental temperature (<20°C) of the sampling sites might have made this region unsuitable for tick adaptation extending the development phase (120 days) at temperatures of 18°C [54]. In addition, a small number of tick samples was collected from one location in the present study. Another limitation is that no livestock sera were collected because of the local cultural customs.
CONCLUSION
This study provides information on the microbial communities of Hy. marginatum in Tibet, China, and provides the first report of R. aeschlimannii in Hy. marginatum in Tibet. The results of this study also indicated that yaks in Tibet are exposed to R. aeschlimannii. Molecular and serology methods are important for investigation of R. aeschlimannii in livestock and farmers with close contact with Hy. marginatum in Tibet.