Introduction
Hypoxic ischaemic injury (HII) is characterized by altered cerebral blood flow and decreased oxygenation resulting in neurologic dysfunction.(1) Clinical manifestations of hypoxic ischaemic injury range from neonatal encephalopathy to seizure disorders and cerebral palsy in older children.(1–3) HII and its clinical sequelae present a global health burden with significant morbidity and mortality in neonates, infants and older children.(4,5) Magnetic resonance imaging (MRI) is recognized as the gold standard in identifying the aetiology of neonatal encephalopathy, identifying patterns of HII and a diagnostic tool for predicting long term neurologic outcomes.(1,2,6,7)
HII is one of the most prevalent aetiologies of cerebral palsy (CP).(3,8–11) In low- and middle-income countries, including South Africa, the estimated prevalence of CP is reported in up to 15.2 per 1000 live births, and between 1.4 and 4 per 1000 births in high-income nations.(10,12,13) MRI is suggested for children with CP after the age of 2 years to obviate the difficulty in interpreting developing myelination and reduce the risk of missing milder injuries.(14,15) MRI abnormalities may be seen in up to 85% to 95% of children with CP, despite 25% to 50% of children with CP having a normal perinatal history and absence of neurological signs in the neonatal period.(7,8,14,16,17)
At present, there is limited published data from low and middle-income countries describing the imaging findings of HII and cerebral palsy in children using MRI.(7,18)
In 2015 a verified analysis of licensed South African diagnostic imaging equipment detailed the disparaging shortage of specialized radiology services, including MRI in the state health sector.(19) In the same year, Johannesburg had 0.3 MRI facilities per million population. Consequently, children in our setting, are imaged following the neonatal period once the diagnosis of developmental delay or cerebral palsy has been established clinically. The surging epidemic of medicolegal action in the setting of birth asphyxia, HII and CP afflicts a growing number of medical service providers. MRI remains an invaluable tool in the assessment of such cases and guiding legislative recourse. Thus, this study aimed to identify the MRI findings in children with suspected HII in a South African population.
Materials and methods
MRI examinations, performed at Charlotte Maxeke Johannesburg Academic Hospital, in Johannesburg, South Africa for children with clinical suspicion of HII, including CP, were retrieved and evaluated. All the MRI studies were acquired using the institution's 1.5T Siemens Syngo system, paediatric brain MRI protocol, including Diffusion Weighted Imaging (DWI). A retrospective analysis was performed for the study period January 2014 to January 2016, wherein137 children were eligible for inclusion. All children younger than 15 years of age who had undergone a MRI brain examination were included where the clinical indication was for: suspected HIE, poor condition or resuscitation at birth, neonatal encephalopathy or altered level of consciousness, cerebral palsy, delayed milestones, seizures, cognitive or visual impairment and focal neurological deficit, including tone, motor, reflex, speech, feeding and movement abnormalities. Exclusion criteria included those with known congenital neurological anomalies, central nervous system tumours, chromosomal abnormalities, neurocutaneous syndromes, head trauma and instances of MRI demonstrating solely congenital structural abnormalities. Prematurity, low birth weight and therapeutic hypothermia were not exclusion criteria.
Included MRI studies were evaluated by three radiologists, (NM, TP, HM) with a minimum of 4-years’ experience reading paediatric MRI brain studies within the South African setting. The imaging readers were blinded to the clinical details (except the age of the child at the time of the MRI to correlate with appropriate milestones) and blinded to each other's imaging findings. Clinical parameters available at the time of the MRI were captured by an individual (LL), who was not involved in the imaging reading process.
A simplified MRI injury pattern (Table 1) described by Charon et al. for neonatal HII and which previously demonstrated good to excellent inter-reader variability was used for MRI evaluation.(20) The imaging readers received a prototype manual outlining specific MRI patterns of injury and included examples of each injury type at various ages of imaging. Standardized myelination tables and graphic examples were referenced to facilitate MRI evaluation precision. A final MRI evaluation was computed using a majority consensus reading (2 out of 3 readers). The study was approved by the University of the Witwatersrand Human Research Ethics Committee.
Description of simplified criteria used for MRI pattern of injury evaluation as described by Charon (20)
Pattern | Criteria |
---|---|
Normal | No signal abnormality in the entire brain. |
Punctate periventricular white matter injuries | Punctate or small, confluent periventricular (<1 cm) white matter signal abnormalities. |
Watershed | White matter (except punctate periventricular) or cortical signal abnormalities in the intravascular boundary zones, without central or diffuse pattern criteria. |
Central | Signal abnormalities in at least one of the following regions: lentiform nucleus, caudate nucleus, thalamus, perirolandic cortex, cerebral peduncle or hippocampus, without diffuse pattern criteria. |
Diffuse/mixed | Diffuse white matter signal abnormalities involving at least four lobes or cortical injuries involving more than the perirolandic cortex or watershed cortex, or watershed and central pattern criteria. |
Statistical analysis using SAS Version 9.2 was performed. Descriptive statistics were calculated for categorical data. Clinical characteristics between the different HII categories were compared using Chi-squared Test. A significance level p-value < 0.05 was used. Inter-reader variability was evaluated using Randolph's Free-marginal multi-rater Kappa.
Results
Of the 137 children evaluated, 63.3% were male. The range of children's ages at the time of the MRI study was 22 days to 12 years, with a mean age of 15 months. One child (0.8%) had an MRI in the neonatal period. Almost half (46.9%) of children were older than 2 years at the time of MRI.
The mode of delivery was available in only 5 children (3.9%); 3 of the 5 (60.0%) children delivered by normal vaginal delivery and 2 of the five (40%) children by Caesarian section. 16 (12.5%) children were documented to have received resuscitation at birth; however, specific details of the events were not available. Perinatal resuscitation data was not available for 109 (85.2%) children.
Children with neurological deficits commonly manifested with a combination of deficits (n=46; 35.9%), including tone abnormalities with comorbid seizures. Description of developmental delay was inconsistent; however, a combination of developmental delay (speech and motor) was most frequently observed, n=35 (27.3%). Among the children with clinical HIE, 3 out of 5 were classified as severe, Supplementary Table 1. Details pertaining to therapeutic hypothermia were not available in the MRI referral, consequently all included MRIs were interpreted with the presumption that this intervention had not been administered.
MRI DICOM data was available for 128 (93.4%) children. Examples of MRI injury pattern types are demonstrated with FLAIR and T2-weighted imaging in Figure 1. A normal MRI from our sample is included for comparison. Complete reader discordance (deficient two out of three majority reading) occurred in 2 of the MRI cases. MRI evidence of HII was present in 54 (42.2%) of MRI studies and 41 (32.0%) children had entirely normal MRI studies, Supplementary Fig. 1.
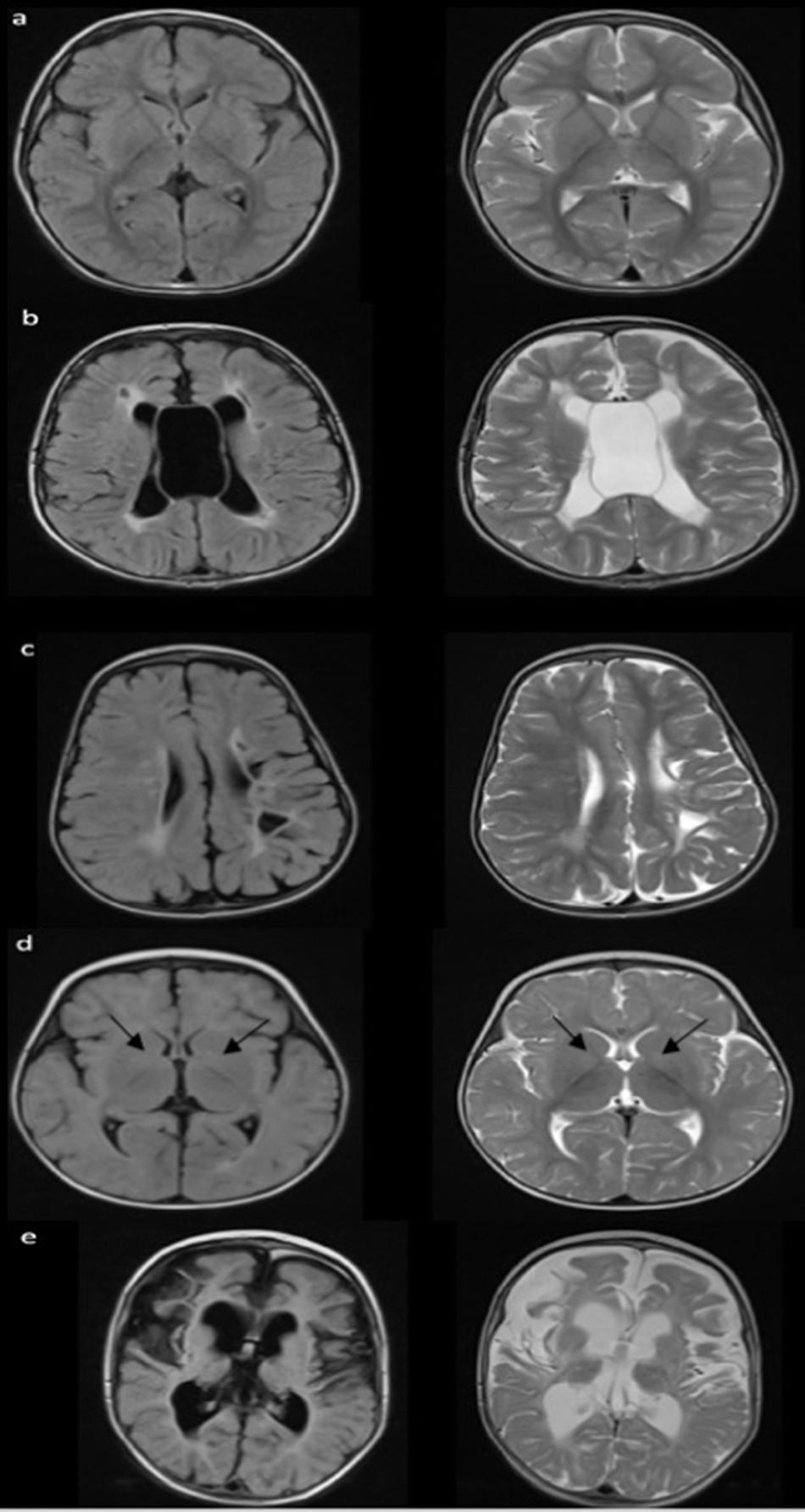
Examples of MRI pattern of hypoxic ischaemic injury with FLAIR (left) and T2-weighted imaging (right). a No identifiable injury, for comparison in a 3-year old child. b Punctate periventricular pattern in a 4-year old child. c Watershed pattern in a 2-year old child. d Central pattern in a 2-month old child, black arrows indicate symmetrical hyperintensity within the globus pallidi. e Diffuse/mixed pattern in a 6-month old child
For miscellaneous MRI findings, including ventriculomegaly, cerebellum, sella and hippocampal evaluations, Kappa values indicate near-perfect inter-reader agreement.
The inter-reader Free-marginal Kappa value for the MRI pattern of injury was 0.30, Table 2.
Description of final MRI findings with inter-reader Free-marginal Kappa n = 128
Finding | Frequency (%) | Inter-reader Free-marginal Kappa |
---|---|---|
MRI pattern of injury | 0.30 | |
Germinal Matrix Haemorrhage | 0.90 | |
Hippocampus | 0.90 | |
Cerebellum | 0.82 | |
Ventriculomegaly | 0.94 | |
Posterior fossa | 0.55 | |
Focal intra-axial lesions | 0.93 | |
SellaNormal | 128 (100) | 0.93 |
Miscellaneous findings |
In children with MRI features compatible with HII, 25 (19.5%) and 30 (23.4%) demonstrated punctate periventricular white matter injuries and diffuse patterns respectively. Watershed and central patterns of injury represented a smaller proportion and included 4 (3.1%) and 13 (10.3%) of evaluated MRI studies, Supplementary Figure 2.
All of the MRI studies which demonstrated ventriculomegaly had some degree of corpus callosum thinning. Corpus callosum dysgenesis was present in 1 (0.8%) MRI without associated ventriculomegaly.
Children with documented gestational age <36 weeks demonstrated a spectrum of MRI patterns of injury. Punctate periventricular white matter injuries were the commonest in this group, as noted in 9 (34.6%, p=0.2677) children. The only child with a documented birth weight <1000 g was found to have a punctate periventricular pattern of injury. Similarly, children with birth weights between 1000 and 2500 g were commonly found to have a periventricular white matter pattern of injury, n=4 (36.4%).
Discussion
In the setting of HII, MRI is a reliable biomarker and provides invaluable insight into the possible causation of neurological deficit and furthermore has the potential to predict specific clinical manifestations.(17,20) MRI classification of HII patterns of injury is the current neuroimaging practice, facilitating standardized lexicon and common understanding between imagers and referrers.(21) The current study demonstrates a spectrum of MRI patterns of injury in children with suspected HII in a South African population.
Our study reiterates the predominant pattern of periventricular white matter injury in premature infants as described previously in the literature.(17,20) Conversely, all the children in our study with documented gestational age ≥ 36 weeks demonstrated neither punctate periventricular nor watershed patterns of injury, thus providing prognostic implications for premature infants and their term counterparts and their expected relative neurologic deficits. Published literature describes a significant correlation of the severity of central grey nuclei injury with the severity of CP, specifically motor deficits.(22,23)
The high cost and limited availability of MRI in the South African state health system is evident in this cohort, where almost half the study cohort were imaged beyond the infant period. State health resources remain deficient with a high disease burden of HIV, TB, non-communicable diseases and trauma.(19) This is compounded by the exponential rise in litigation claims relating to HII and CP, invariably resulting in an irreparable cycle of limited funds, access to adequate neuroimaging and inability of the state to confidently defend such cases without radiological evidence.(11,21) The imbalance of MRI availability is detailed by Kabongo et al where in Gauteng province in 2015, there were 46-fold more MRI facilities in the private sector and interestingly 30-fold and 70-fold more in the United Kingdom and Organisation of Economic Cooperation and Development (OECD) during the same period respectively.(19) This wide discrepancy in access and demand of advanced imaging will further challenge any anticipated state healthcare reform. Almost a third (32%) of MRIs in our study were normal and 26% were abnormal without evidence of HII. The relevance of these two groups, combined making up greater than 50%, is paramount in providing sufficient proof to mitigate potential malpractice claims and refuting HII. This is despite the delayed median imaging time from suspected age of injury. Similar inferences were described by Elsingergy et al in a review of 1620 MRIs of South African children with CP involved in medicolegal cases, where the median interval of suspected injury to imaging was six years. Their study established an MRI diagnosis in 92%, but only 76% of cases of CP demonstrated MRI evidence of HII and 31% were either non-HII findings or preterm pattern of HII which can be successfully defended in malpractice suites.(8) The greater percentage of abnormal MRIs (92% vs 68%) and those consistent with HII (76% vs 42%) in Elsingergy et al analysis, compared to our cohort is explained by selection bias; in our study the inclusion criteria were expanded to include any child suspected of HII, including the subset of CP.
Inter-reader free-marginal agreement for MRI pattern of injury was poor with a Kappa value of 0.3. Possible explanations include subjectivity of the MRI pattern of injury classification with the potential overlap of pattern types, specifically punctate periventricular white matter injuries and watershed patterns. Improved inter reader agreement may in the future be established with comprehensive injury pattern reference guides including a range of children ages. Standardized referral templates for suspected HII MRI studies will facilitate clinical contextualization.
Limitations
Limitations of our study include an incomplete clinical data set, specifically gestational age, birth weight, perinatal adverse events and standardized clinical assessment. The explanation for this is multifactorial; where more than 20% of children were referred for MRI from a satellite hospital and majority of children were imaged at older than 24 months and perinatal details were incomplete. Despite a positive correlation in our study of premature infants and periventricular white matter injuries as described in the international literature, accurate perinatal data could have improved significance of our findings.
Assessment of HII in the neonatal period and imaging of the sequalae of possible HII in older children illustrates a dichotomy of disease. Our study had a single child imaged in the neonatal period and highlights the local resource deficit regarding pediatric MRI.
Conclusion
Hypoxic ischaemic injury and cerebral palsy continue to cause significant morbidity in the South African paediatric population. In this study, we describe a spectrum of MRI injuries in children with suspected hypoxic ischaemic injury. Periventricular white matter pattern of injury was the most common type in premature infants, congruent with international cohorts. Despite the limited availability of MRI in the state health sector, delayed neuroimaging in the setting of suspected HII may provide pivotal evidence of causation. We recommend future studies with robust, standardized clinical criteria and a validated MRI injury reporting scale.