INTRODUCTION
Osteoarthritis (OA) is the most common joint disease in humans, and is increasingly becoming one of the major health problems worldwide. Predominantly it is a clinical, social and economic problem associated with ageing. In addition, it also represents a major public health concern for young individuals, including athletes and those suffering from extreme weight problems. OA is characterised by degenerative changes with regard to the composition, organisation and mechanical functions of the articular cartilage, subchondral bone, and other joint tissues (Nesic et al., 2006; Bradley et al., 2007).
In biological tissues, quantitative mapping of the collagenous scaffold of tissue extracellular matrix (ECM) can be achieved using microbeam coherent small-angle X-ray scattering (cSAXS). The present work concerns the organisation of collagen in the hyaline cartilage and subchondral bone of articulating joints. The collagenous component of ECM accounts for about two-thirds of the dry weight of adult articular cartilage, the load-bearing capacity of the joint, which depends on the organisation of the scaffold making up the zonal changes in the fibrillar architecture with tissue depth. In injury (for instance, as in OA), there would seem to be little capacity for repair of the overall collagen architecture. The structure of an adult articular cartilage can be depicted schematically as being arranged in four zones: superficial, intermediate, deep, and a calcified zone (Fig. 1), based on the organisation of chondrocytes, orientation of collagen fibres, and distribution of proteoglycan. The four zones of articular cartilage, visible under light microscopy, but whose bulk organisation can only be made apparent through the microbeam X-ray diffraction (μXRD) from a synchrotron cSAXS beamline, are as follows: (i) the superficial or tangential zone; (ii) the intermediate, transitional zone; (iii) the deep or radial zone; and (iv) the calcified (perhaps better referred to as calcifying) zone, mineralised and separated by the other non-mineralised layers by a distinct border known as the tidemark. These zones differ in their collagen orientation; the predominant type of collagen is collagen-II, although the collagen phenotype of the calcifying zone of cartilage forming the interface just below the tidemark also includes other forms such as type X collagen.
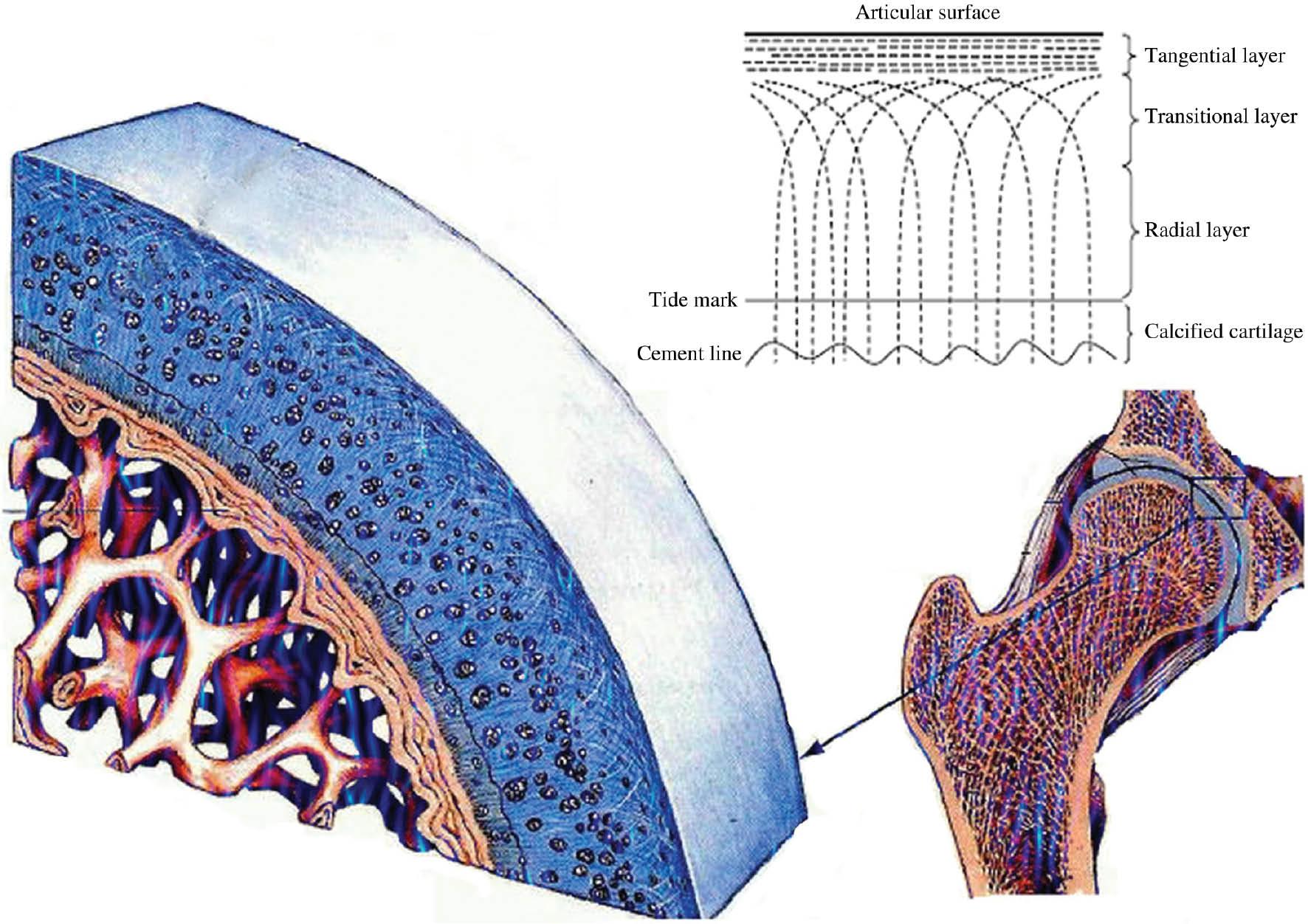
Collagen fibres laid down parallel to the articulating surface, with gradual reorientation of the fibres until they are approximately normal to the subchondral bone.
As shown in Figure 1, the bone–cartilage interface region has within it a calcified cartilage region separated from non-mineralised cartilage and bone (Gupta et al., 2005), delineated above by the tidemark and below by the cement line. The calcified layer is mineralised and separated by the other non-mineralised layers by a distinct border known as the tidemark. The particular zone between the tidemark and the cement line is considered to be a zone of considerable enzymatic activity. As a biomechanical entity, the bone–cartilage interface transfers stress from the more elastic (soft) matrix of cartilage to the significantly harder underlying bone (Farkasja and Synecek, 1965; Fratzl, 1996), but there remains very little detailed information available about the elemental distribution of this region.
The purpose of the present investigation was to perform orientational mapping over selected areas encompassing the bone–cartilage interface in a slice of human femoral head, using the cSAXS technique. The remainder of this paper describes the experimental method, the main results and the outcome of the study.
Identification of type X collagen suggests its involvement in regulation of mineralisation at this critical interface. The expression of growth plate-like properties would allow the deep chondrocytes of mature articular cartilage to play a role in remodelling of the joint with age and in the pathogenesis of OA. The cartilage acts hydrodynamically to protect the integrity of the chondrocyte and its pericellular microenvironment during compressive loading.
Collagen-X is normally restricted to the thin layer of calcified cartilage that interfaces articular cartilage with bone. Type X collagen is a short-chain, non-fibril-forming collagen with a restricted tissue distribution within the hypertrophic calcifying region of growth plates in fetal and developing bone, where it makes up 45% of the total collagen. It has been proposed that type X collagen may play a role in regulating the mineralisation of cartilage calcification; however, this remains to be proven. The present paper is concerned with orientational mapping of the surface region of diseased femoral head following total hip replacement surgery. The tissue shows visible manifestation of OA and non-malignant bone disease.
The articular cartilage is a dynamic and highly organised connective tissue composed of chondrocytes and ECM. The ECM provides for the unique biomechanical capacity of the articular cartilage, while the chondrocytes are responsible for synthesis and degradation of the ECM, growth factors and enzymes that control the turnover of the ECM (Lai et al., 1991). These enzymes play an important role in the normal process of growth and remodelling as well as in the degenerative and inflammatory processes of arthritis. Their function relies on the presence of divalent cations such as zinc and calcium. In addition, other enzymes such as alkaline phosphatase are involved in cartilage mineralisation and their functionality also relies on the presence of metallic cofactors such as calcium and zinc. The local concentration of divalent cations is, therefore, of great interest in cartilage pathophysiology.
THE PSI CSAXS BEAMLINE
Among the most salient tools at the cSAXS beamline at the Paul Scherrer Institute (PSI) synchrotron source is the PILATUS II detector, a PSI-developed two-dimensional hybrid pixel array detector operating in single-photon counting mode. The PILATUS detectors are large-area, single-photon counting hybrid pixel detectors. They are in part designed for biomedical applications, making use of X-ray diffraction at synchrotron light sources. With 2.5 × 106 pixels covering an active area of 25.4 × 28.9 cm2, the PILATUS 2M detector, installed at the cSAXS beamline, comprises 24 modules mounted on a precision frame. The main feature of the detector is its dynamic range of 20 bits, that is, more than 1 million X-rays can be stored in each pixel. A massive parallel readout of the detector results in a readout time of 3.6 ms and a frame rate of 12 Hz. Other important features are the narrow point spread function, the absence of electronic noise, an electronic shutter, and the possibility of suppressing fluorescent background from the sample. The pilot phase of user operation at cSAXS began in July 2007, wherein the present series of investigations formed one of the pilot projects. Normal user operation began in the beginning of 2008. The full experimental details will be published elsewhere.
cSAXS measurements
SAXS measurements were carried out in August 2007 at the PSI synchrotron beamline cSAXS. A monochromatic photon energy of 12.4 keV was used along with a beam of rectangular dimensions of 20 × 5 μm (h × v), with a sample-to-detector distance of 2.1 m. The slice was mounted on a motorised sample stage to allow the alignment and orientation of the sample to the beam. Subsequently, raster scanning was performed in the horizontal and vertical directions, covering an area of up to several square millimetres to encompass the cartilage surface and underlying bone. The scans were made for a range of momentum transfer values to include the prevalent d-spacings of the predominating collagen types of collagen and bone. The two-dimensional X-ray scattering patterns were acquired using a PILATUS 2M detector with a dwell time of 1 s per point, with individual scans taking periods of between a few hours up to 8 h. Before scanning, camera length calibration of the set-up was performed using a silver behenate powder standard.
Slices of human femoral head were performed covering an area of 1 mm × 1.5 mm, from the cartilage surface through to the underlying bone (Fig. 2), by which a sequential series of diffraction patterns were obtained.
RESULTS AND DISCUSSION
Figure 2 shows scattered intensity images on a log scale in reciprocal space from cSAXS scans from a human femoral head at different locations on the bone.
The middle part of Figure 2 shows a picture of the bone–cartilage sample with locations of four scanning areas labelled as s1, s2, s3 and s4. The top row of Fig. 3 shows what we called combined scans with different q-spacing values between 2.5 and 78.1 nm. The combined scans are formed from symmetric and asymmetric intensity mapping together with the orientation maps. The collagen orientation in this broad collagen integration range is very apparent.
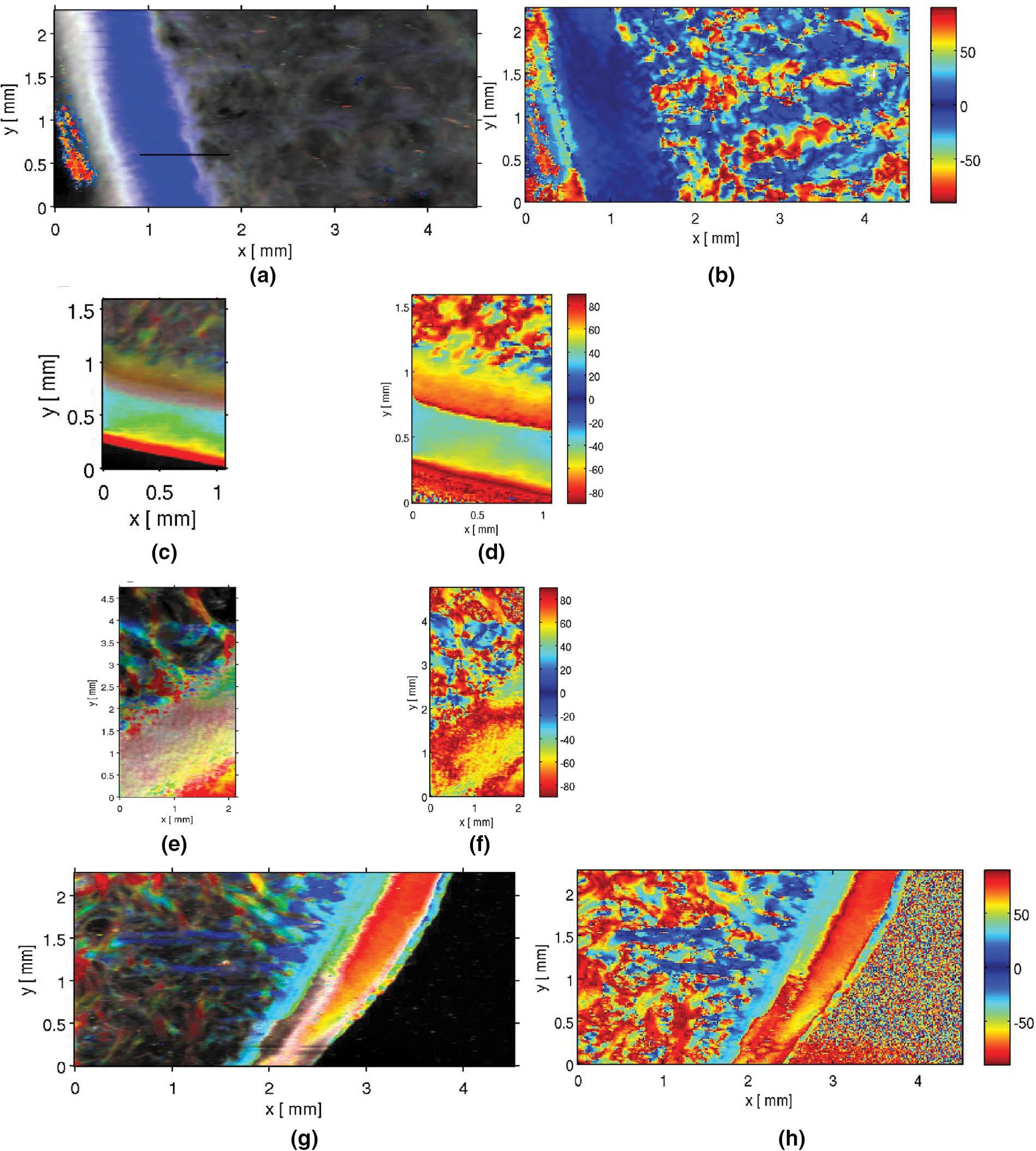
cSAXS results from four different scanning areas shown schematically on the picture of the bone–cartilage sample in the middle for 2.5–78.1 nm spacing. (a, b) Combined intensity mapping and orientation mapping for s1. (c, d) Combined intensity mapping and orientation mapping for s2. (e, f) Combined intensity mapping and orientation mapping for s3. (g, h) Combined intensity mapping and orientation mapping for s4. Abbreviation: cSAXS, coherent small-angle X-ray scattering.
However, Figure 4 shows orientation maps at narrower collagen integration ranges. It is apparent that while we can see collagen type-X in one plot, we lose it at other ranges; these orientation maps provide invaluable information on the collagen spacing, including their identification.
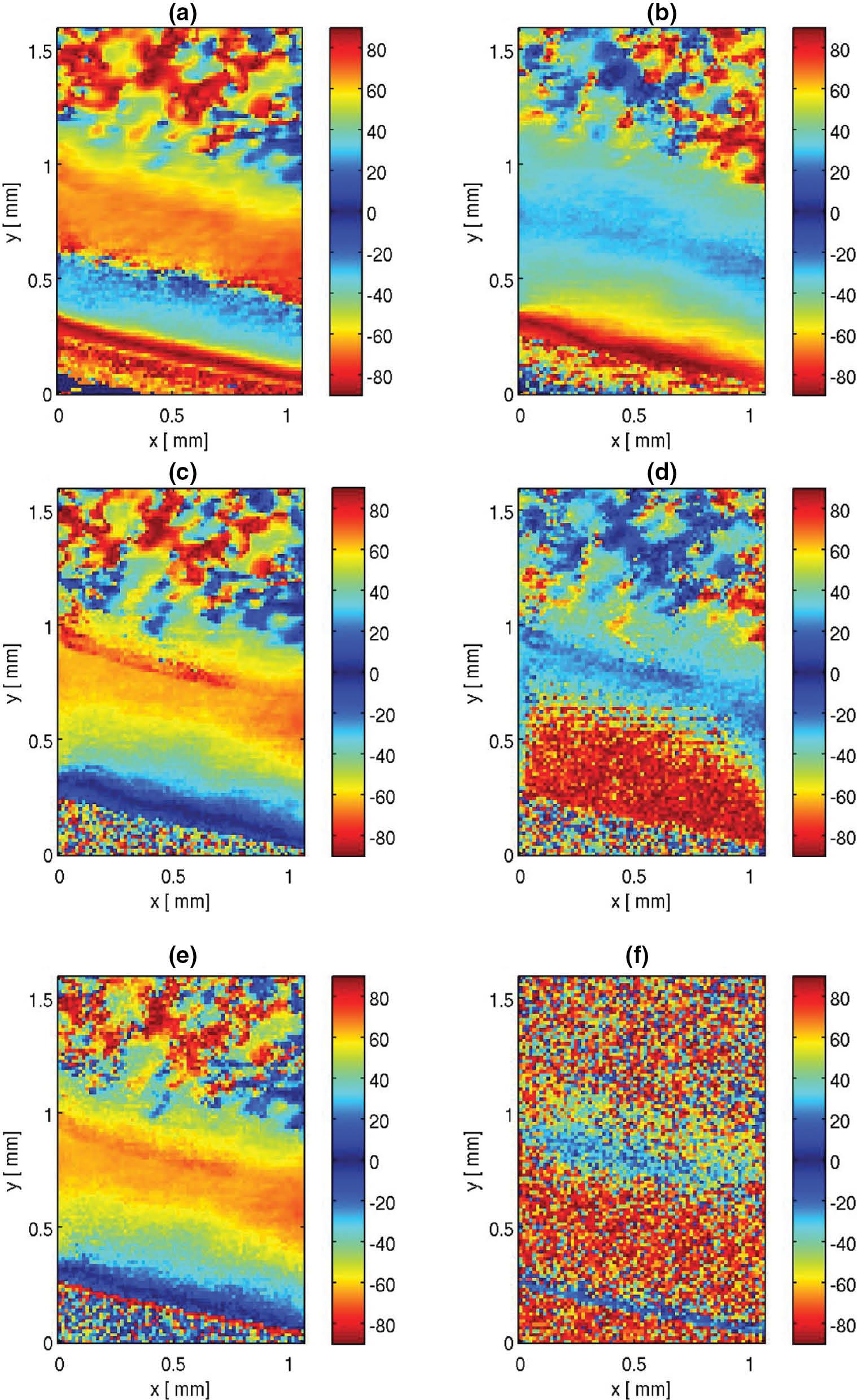
Orientation scans differentiated only by the choice of k-space for the scan area s2: (a) 52.1–78.1 nm, (b) 25.0–46.3 nm, (c) 20.2–24.0 nm, (d) 16.9–19.5 nm, (e) 11.8–13.9 nm and (f) 10.6–11.5 nm. It is apparent that by changing the k-space interval the collagen-X is no longer visible in Figure b.
These maps exhibit a rather rich set of features, which is seen as a repeating orientation from parallel to the surface of the articular cartilage, reorienting to approximately 90 degrees and then reorienting again in the deeper part of the cartilage to accept the force onto the bone. If the q-spacing is changed, this second repeating unit disappears and this unit is thought to be collagen-X, which is a network-forming collagen and clearly of different spacing from the fibre-forming collagen-II above it. It is also possible to have combined mappings of these bone–cartilage areas. The map between 83.3 and 96.2 nm, and 25.0 and 46.3 nm is virtually the same in all the scanning regions, suggesting that the signal in the high q-region or range is related to a broader peak that sits below the collagen peak and is visible on both sides of it. However, from the combined plots, one can see fine collagen fibres that are only visible in the 83–96 nm range.
Figure 5 shows plots of intensity against q-values. The angular segments were formed on the diffraction patterns as shown in Figure 2 and averaged along a certain line segment. The intensities are different depending on the collagen orientation. For cartilage in the region s1, a broad cartilage region spacing of about 64–65 nm is seen, whereas the integration across some fibres in the bone gives a shorter length of 63 nm, plus a rather broad peak of 4.2 nm. This peak is a characteristic spacing of the triple alpha helix making up collagen reportedly coming from the proteoglycans, a highly charged proteinaceous structure within the cartilage that retains water as this signal coincides with neither the collagen fibres in the bone nor in the cartilage. This peak is also observed for the scan area s3. For the scan area s2, there is a clearly defined small outer region that exhibits a larger spacing than the rest. The collagen fibre bundles in the bone seem to have in general much smaller spacing than the cartilage region, but different values have been obtained. The outer edge of the cartilage region has a large spacing of about 66 nm. For the scan area s3, we see loop structures that seem to be corresponding to the trabecular region, with structures shorter than 64 nm, and a 4.2-nm broad peak is also visible in the bone line scan. The average line scan results for the scan area s4 show 64–65 nm in the cartilage region, whereas the integration across some fibres in the bone gives a shorter length of about 62–63 nm. At the bone–cartilage interface, asymmetric peaks are present with peak values of 65–66 nm with a broad shoulder to higher q-values corresponding to a distribution of smaller spacings (in addition to the main components).
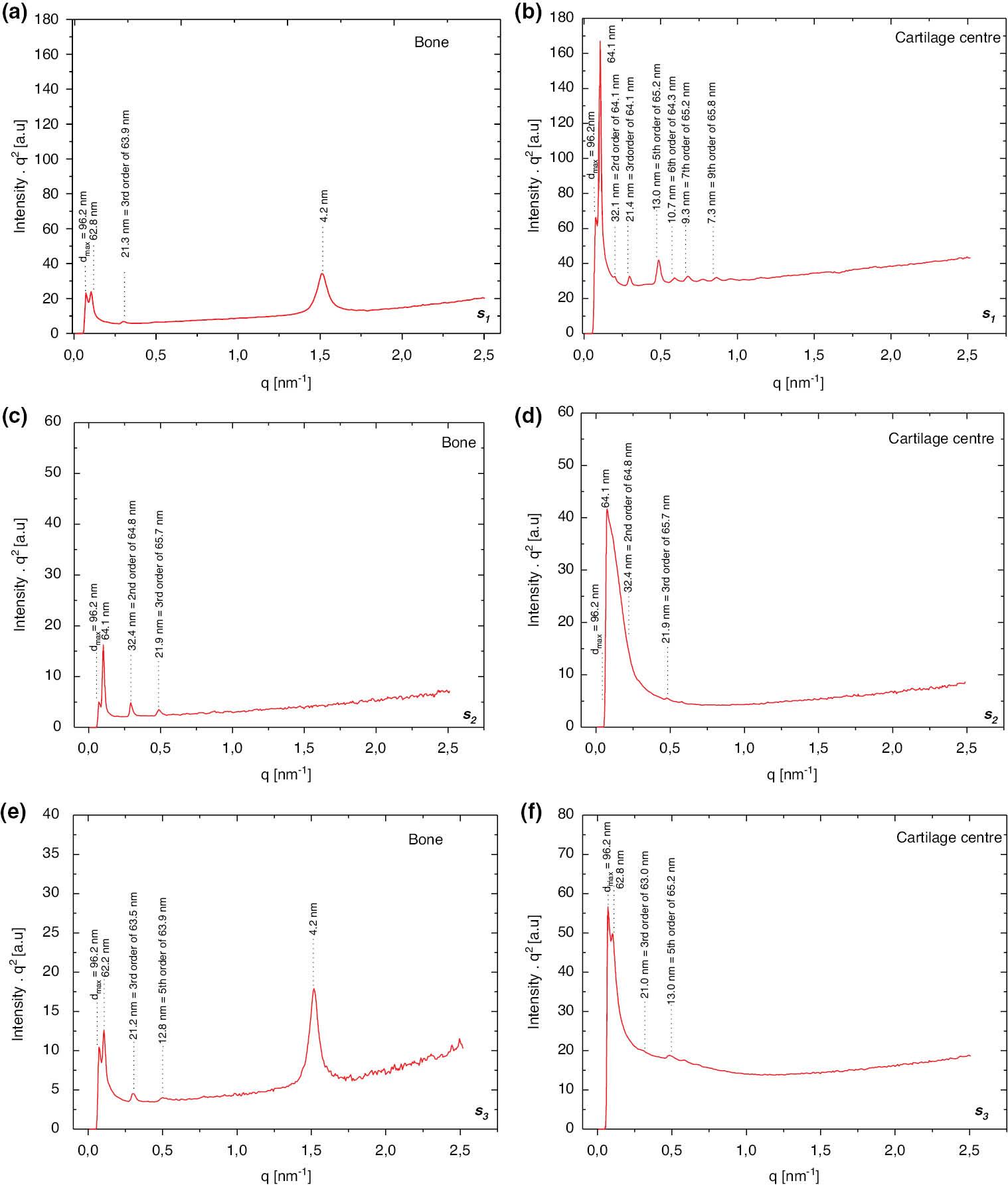
Intensity versus q-plots for three scanning areas along a line either in the bone or at various locations in the cartilage.
Figure 6 shows detailed averaged line plots in the bone, the inner part of the cartilage, the central part of the cartilage and the outer part of the cartilage. We see a broad peak corresponding to 44.6 nm and no other collagen peaks. This peak does not correspond to any of the recognised or known collagen-type spacing, and to the best of our knowledge this is the first time it is being observed. At the cartilage centre, a peak of 66 nm as well as a tail corresponding to shorter spacing are seen. The collagen fibre in the bone has a shorter spacing of 62–63 nm than the broad cartilage region. The centre of the cartilage region has about 66-nm spacing.

Averaged line scans for the scan area s4, corresponding to the line chosen in the bone, inner part of the cartilage, central part of the cartilage and outer part of the cartilage.
The results so far have presented rather interesting features on the bone–cartilage system. While the scan for the area s4 shows broad and thick collagen fibre bundles in the bone region, scan s1 gives thin fibres. The outer cartilage looks rather different and area s1 seems to have two regions (inner and outer cartilage), whereas s4 seems to have a central region in the cartilage and two outer stripes (i.e. towards the bone and in the outside region). It is also interesting to note that the high q intensities follow more of a q -1 instead of q -2 law, which may be indicative of a different shape of the scatterers.